Corals: Ocean engineers are under threat
PDF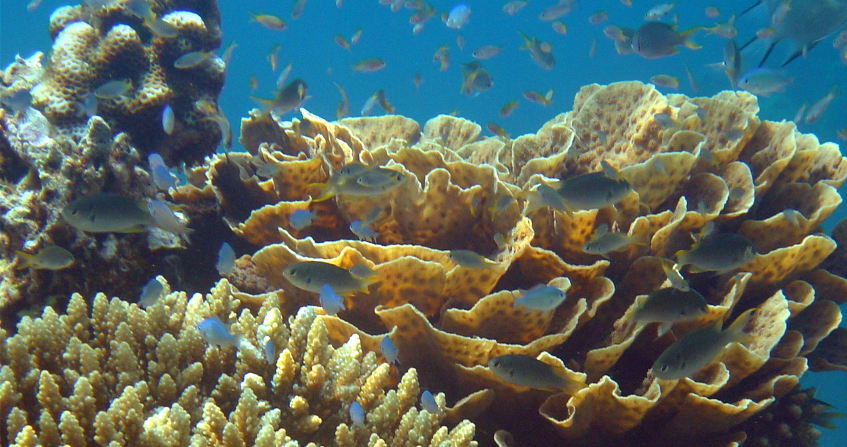
Coral reefs are one of the most complex and richest ecosystems on our planet. They are based on a bioconstructed mineral (or biomineral) structure built by corals that provides ecological niches for many species that find food and protection there. The success of coral reefs is based on the symbiosis established by the coral (host) with unicellular algae of the genus Symbiodinium (zooxanthellae) which allows life in tropical waters very poor in nutrients. This mutualistic symbiosis has required the development of adaptation mechanisms between the two partners, forced to adjust to their mutual needs. Despite these adaptations, the balance of the symbiosis is fragile and strongly relies on environmental conditions. When the coral is subjected to high pressures such as high temperatures, the balance can be disrupted and the zooxanthellae expelled by the host, causing the coral to bleach and eventually leading to its death. Currently, there are many threats to coral reefs health and the symbiotic balance of corals. On a global scale, reefs are subject to physical and chemical changes in their environment due to global warming and ocean acidification. In addition to these changes, there are local pressures related to human activities such as pollution, overfishing and unsustainable tourism. Despite the adaptive capacities of corals, observations and models predict that more than 90% of the world’s reefs will be affected by major bleaching episodes by 2050. These figures demonstrate the urgency and need for action to preserve this unique ecosystem, which is essential to the economies of many countries.
1. Coral reefs: biodiversity hot spots
1.1. Formation, distribution and importance
Coral reefs represent about 0.16% of the seabed (about 600,000 km2) and 10.8% of the coastal bottom. Despite their small areas, reef ecosystems are major ecological reserves that are home to about 30% of the marine biodiversity known to date [1] including ¼ listed fish species [2]. They are defined as the richest marine ecosystem in terms of biodiversity and are often compared to their terrestrial equivalent: the tropical forest.
Beyond their ecological importance, coral reefs provide important ecosystem services to humans. Bordering the coasts of more than a hundred countries, they play an essential role for their populations. Their extreme biodiversity contributes to the economic development of these countries, through fishing activities, tourism and provision of building materials. They also play a role in protecting coasts against erosion, storms and cyclones [3]. In total, more than 500 million human lives are directly linked to the services provided by coral reefs. The net benefits provided by coral reefs (taking into account fisheries, coastal protection and biodiversity) vary according to estimates from $30 billion per year [3] to $375 billion per year [4].
1.2. Reef functioning, the key role of coral
Beyond its role as a framework, coral is also a source of nutrition in the reef. Indeed, many fish species graze coral and are therefore referred to as corallivores (Figure 2B,C). This is the case, for example, for some damselfish, gobies, butterfly fish or parrot fish.
In addition, coral is also a major source of organic matter in the reef through mucus excretion. This mucus, composed mainly of carbohydrates and proteins, is then dispersed in the water column and can be used by other reef organisms (Figure 2D). Organic matter excreted by coral is essential to the functioning of the reef because it supports benthopelagic production (production in benthic areas, at the bottom of the sea, or pelagic, in the open ocean) and participates in the recycling of essential elements (nitrogen, phosphorus…) in the very oligotrophic water [5] of the reef [6].
2. Reef-building corals
2.1. Scleractinian corals, symbiotic animals
Reef-building corals, or corals of the order Scleractinian belong to the Cnidaria branch, which also includes jellyfish, sea anemones or gorgonians. Appeared in the Precambrian, the oldest fossils identified are dated to -700 million years ago. The “modern” reefs appeared in the Triassic period about 200 million years ago, probably as a result of the development of a mutualist association (symbiosis) between coral and a type of unicellular algae commonly known as zooxanthella (see Symbiosis and parasitism).
When they were first described, it was thought that the algae associated with coral, zooxanthellae, all belonged to a single species, Symbiodinium microadriaticum. The development of molecular analysis techniques has made it possible to demonstrate that they constitute a genus, Symbiodinium, containing a large complexity of species or subspecies that has not yet been resolved in terms of taxonomy [7].
The symbiosis between coral (host) and zooxanthellae is mutualistic because both partners benefit from the association (developed below). Zooxanthellae are actually integrated into the host cells (see Figure 3E), this is called intracellular endosymbiosis. Each coral contains an average of 1 million zooxanthellae per cm2 of tissue surface area. This density is remarkable and is largely responsible for the colour of the coral.
2.1.1. Benefits of symbiosis
The role of zooxanthellae for coral is mainly trophic. Indeed, by providing a variety of carbon compounds (in the form of carbohydrates, amino acids and lipids) to the host, photosynthesis of zooxanthellae is a major source of carbon for coral. Most of the products of photosynthesis (75-95%) are thus transferred to the host, which uses them mainly for its own respiration and metabolism, as well as for mucus production. Zooxanthellae can thus contribute up to 100% of the host’s energy needs. Coral thus passes from a heterotrophic organism status (use of organic carbon sources via heterotrophic nutrition) to a mixotrophic organism status (use of both organic carbon via heterotrophy and inorganic carbon via zooxanthellae photosynthesis [8]).
Inherent to the photosynthetic properties of zooxanthellae, the oxygen produced by photosynthesis is an important source for host’s (and of course its zooxanthellae) respiration. Finally, zooxanthellae participate in defences against harmful light radiation (such as ultraviolet radiation) by producing key defence molecules such as Mycosporin-like amino acid (or MAAs), true sun filters.
For zooxanthellae, living in an association also has many advantages. Due to their intracellular location, zooxanthellae benefit from an environment protected from grazing and the stability of the animal cytoplasm. Zooxanthellae also benefit from nitrogen and phosphorus from the recycling of the host’s metabolic waste and inorganic carbon (CO2) produced directly by animal metabolism [9], thus avoiding any unnecessary loss in a generally nutrient-poor environment. Nevertheless, to ensure optimal photosynthetic activity, coral actively participates in the supply of CO2, nitrogen and phosphorus by absorbing these compounds from the external environment.
Thus, coral symbiosis is an association that benefits both the host, which acquires a new metabolic capacity, and zooxanthellae, which benefit from a stable environment and constant access to the elements necessary for their metabolism.
2.1.2. Costs of symbiosis
Despite its important advantages, symbiosis also imposes a cost on both partners and leads to co-evolution processes to limit them. For example, because of its light requirements for photosynthesis, the host is forced to develop only in the euphotic zone where exposure to high light intensities can cause significant oxidative stress. In addition, most of the photosynthesis produced by zooxanthellae is transferred to the animal host, which is a loss much greater than the 5% lost in the environment by free algae. Finally, the oxygen supply from zooxanthellae is certainly a metabolic advantage, but it is also a chemical constraint caused by the consequences of strong tissue hyperoxia leading to an increased production of reactive oxygen species. The symbiosis has thus been accompanied by an increase in antioxidant defences (such as enzymes such as superoxides dismutases) that make it possible to counter its harmful agents.
2.1.3. The coral, a holobionte
If the phototrophic symbiosis between zooxanthellae and coral remains the most well known, we now know that coral also hosts many other organisms: bacteria, archaea, protists, fungi, viruses, all of which form a functional community called holobionte. The microorganisms constituting this microbiota are distributed in communities that develop in different compartments of the coral with particular physicochemical conditions. There are distinct communities between tissues, mucus, skeleton and gastro-vascular cavity [10]. Their role, still little known, seems varied and depends again on the compartment in which they are located. For example, in mucus, ciliates prevent the fixation of parasitic organisms by creating a surface current of about 2 mm.s-1. Bacteria associated with mucus have a protective role against other pathogenic bacteria or viruses [4] and participate in the recycling of nutrients in the holobionte by degrading the organic matter excreted by the coral. In tissues and skeleton, cyanobacteria, or other diazotrophic bacteria, carry out the fixation of dinitrogen, constituting a potential source of nitrogen for the host and Symbiodinium. This nitrogen source as well as sugars synthesized by photosynthesis of bacteria capable of fixing atmospheric nitrogen (called diazotrophs) can be of great importance for the survival of the holobionte under bleaching conditions.
2.2. From coral to reef, biomineralization
The physical structure of coral reefs is formed by the polyps of corals that carry out calcification, i.e. they synthesize a calcareous skeleton from the Ca2+ and HCO3– ions of seawater. This process is called biologically-controlled mineralization or biomineralization [11]. Coral skeleton consists of the organized superposition of layers of aragonite crystals (a form of crystallization of calcium carbonate CaCO3) inserted into an organic matrix consisting mainly of proteins rich in acid amino acids and phospholipids. The organic matrix allows the coral to exercise biological control over calcification, imposing skeletal morphology from nanoscale to centimetre scale.
Corals have a wide phenotypic plasticity explained by the strong influence of environmental parameters on skeletal morphology. Among these parameters, light plays a key role. Yonge & Nicholls [12] had observed that light was essential for the formation of reefs and that this link could depend on the photosynthesis of zooxanthellae. This process is now known as the process of Light-Enhanced Calcification (LEC): in light, the calcification rate is multiplied by a factor of 3 on average, but this factor can reach extreme values of 127 depending on environmental conditions and species [12],[13]. This stimulation takes place as soon as light appears: in laboratory conditions, for example, 25 minutes after the lamps are switched on, the calcification rate of the coral changes from its night value to its day value. The mechanism(s) underlying this relationship remains much debated.
Zooxanthellae could promote calcification by providing the energy and oxygen necessary for its realization as well as precursors for the synthesis of the organic matrix. By absorbing the carbon dioxide required for photosynthesis, they could also promote an increase in pH in the animal’s tissues, thus creating favourable conditions for calcification. Finally, by absorbing some toxic compounds such as phosphates, they could reduce calcification inhibition. It should be noted that these different hypotheses are not exclusive and that several may coexist [10].
3. Corals, organisms in a changing environment
3.1. Global warming and coral bleaching
The increase in ocean temperature, linked to global warming, favours bleaching episodes whose magnitude and frequency increase [14]. In 2016, for example, surface temperature anomalies of up to +3°C in some regions were recorded and caused severe episodes of coral bleaching around the world. As recently as 2017, massive bleaching affected the Great Barrier Reef.
Temperature is an essential environmental parameter because it controls the metabolism and growth of corals. Indeed, most of the body’s chemical reactions are catalysed by enzymes whose activity depends mainly on temperature. Enzyme activity is minimal at low temperatures and then increases to a temperature optimum above which it is then inhibited. Therefore, photosynthesis, respiration and calcification increase linearly with temperature, to an optimum that very often depends on the species studied, before decreasing again.
The boundary between the beneficial and harmful effects of temperature is narrow. Indeed, in addition to inhibiting metabolic enzymes, temperature can, above a certain value (variable according to coral species), alter photosynthetic activity and produce excess free radicals, increasing oxidative stress [15] in cells.
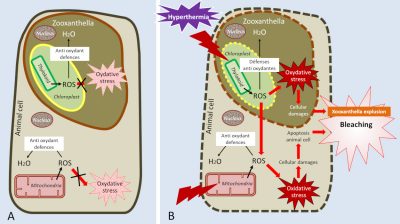
To limit the damage caused by thermal stress, corals synthesize chaperone proteins, called heat shock proteins (HSP), which protect cells from the effect of stress and maintain the function of the proteins with which they are associated. For example, they protect the function of electron transporters from photosynthesis and respiration, thus limiting oxidative stress.
As a last resort, when thermal stress is too high and corals are no longer able to contain oxidative stress, they massively expel zooxanthellae, leading to massive coral bleaching (or mass bleaching event). If stress persists, the amount of symbionts is no longer sufficient for the coral to maintain its primary functions and the colony dies (for a summary of temperature effects, see Figure 5). Too high a temperature is therefore responsible for bleaching and high mortality on reefs [16].
3.2. Ocean acidification
While a large part of anthropogenic carbon dioxide accumulates in the atmosphere, causing global warming as a result of the greenhouse effect, some of it dissolves in the oceans. This dissolution induces a chemical modification of the marine environment called ocean acidification, or the other effect of CO2. Indeed, by dissolving in the oceans, carbon dioxide causes a decrease in the pH of seawater depending on the reaction:
CO2 + H2O ↔ HCO3– + H+ (reaction 1)
(where HCO3– is bicarbonate and H+ is hydrogen acid ion)
The acidity of the oceans has thus increased by 30% since the beginning of the industrial era (pH drop from 8.2 to 8.1, which could reach 7.8 at the end of this century), while the pH has remained virtually unchanged over the past 30 million years. Ocean acidification causes a change in carbonate chemistry, a complex chemical system that plays a major role in the pH balance of seawater and all biological fluids as well as in the formation of sedimentary rocks. Indeed, carbonate ion (CO32-) is the essential brick for the formation of shells and other invertebrate skeletons, including the skeleton of corals when it reacts with calcium (reaction 2) to form calcium carbonate (CaCO3 or limestone):
CO32- + Ca2+ ↔ CaCO3 (reaction 2)
When the acidity of seawater increases, the protons (H+) produced in excess will react with the carbonate ion according to reaction 3:
H+ + CO32- ↔ HCO3– (reaction 3)
Thus, ocean acidification reduces the concentration of carbonates in seawater while increasing the concentration of bicarbonates. This reduction has long been thought to be the cause of the decrease in calcification rates observed in the laboratory or in the field under acidified conditions. It is now known that the main mechanism responsible for the biological effects of ocean acidification is the decrease in pH by itself. Indeed, pH is a key parameter in the physiology of organisms since it regulates many cellular processes, including the activity of many proteins.
Biological effects of ocean acidification include decreased calcification and disruption of physiological processes such as reproduction, nutrition, etc.
However, studies show a wide disparity in the sensitivity of organisms to ocean acidification, particularly in corals. The study of coral reefs exposed to natural sources of carbon dioxide, for example in Papua New Guinea, shows that massive corals resist well, at least up to a pH of 7.7, while branchy corals are very sensitive [17]. If it appears that there will be winners and losers in a more acidic world, the morphology of the reef will be strongly disrupted by the decrease in coral biodiversity and an increase in algal cover [17].
In addition, laboratory studies show that although some coral species may appear resistant to acidification, as their length growth is not reduced, the skeleton of these colonies is more porous, making the branches more fragile and suggesting a dark future for coral reefs.
3.3. The challenges of the current situation: between anxiety and hope
Recent observations and models that take into account global warming predict the loss – or significant alteration – of 90% of reefs by the middle of the 21st century if global warming is limited to 1.5-2°C by 2100 (target set at COP21 [18]). In addition, ocean acidification and other environmental factors such as ocean eutrophication increase the negative effects of global warming.
However, recent studies have shown the potential for physiological acclimatization of corals to strong temperature anomalies. For example, some species are able to modify their zooxanthellae population and regulate gene expression to strengthen defence mechanisms and optimize their resistance to high temperatures. Others are able to compensate for the decrease in photosynthesis by increasing their heterotrophic nutrition. However, this degree of resilience remains low and limited, and coral adaptation/acclimation capacities remain highly debated.
Alarming predictions about the future of coral reefs have triggered an awareness for the need to act protecting them. Scientists, non-governmental organizations (NGOs) and local authorities must work together to preserve this heritage. The effective protection of coral reef biodiversity depends, among other things, on our ability to reduce our impact on the environment and on improving the living conditions of local populations so that they can sustainably manage the resources of the ecosystems on which they depend.
References and notes
Cover image. [Source: © Joël Courtial]
[1] Porter and Tougas (2001, In Encyclopedia of Biodiversity) estimate that 93,000 species are described in reefs out of a total of 274,000 known marine species.
[2] Allsopp, M., Pambuccian, S.E., Johnston, P. & Santillo, D. State of the World’s Oceans. (Springer Science & Business Media, 2008).
[3] Cesar, H.S.J. Coral reefs: their functions, threats and economic value. (2002).
[4] Costanza, R. et al. The value of the world’s ecosystem services and natural capital. Nature 387, 253-260 (1997).
[5] An oligotrophic environment is one that is poor in the nutrients necessary for the growth of marine organisms.
[6] Bythell, J. C. & Wild, C. Biology and ecology of coral mucus release. J. Exp. Mar. Organic. School. 408, 88-93 (2011).
[7] The genus Symbiodinium is now divided into 9 large phylogenetic groups, or clades, named A to I. Each of these clades is itself composed of many sub-classes, now estimated at more than 250.
[8] Houlbrèque, F. & Ferrier-Pagès, C. Heterotrophy in tropical scleractinian corals. Biol. Rev. 84, 1-17 (2009).
[9] Smith, D.C. & Douglas, A.E. The biology of symbiosis (Edward Arnold (Publishers) Ltd., 1987).
[10] Bourne, D.G., Morrow, K.M. & Webster, N.S. Insights into the coral microbiome: underpinning the health and resilience of reef ecosystems. Annu. Microbiol. 70, 317-340 (2016).
[11] Tambutté, S. et al. Coral biomineralization: from the gene to the environment. J. Exp. Mar. Organic. School. 408, 58-78 (2011).
[12] Yonge, C.M., Nicholls, A.G. Yonge, M.J. Studies on the physiology of corals. 1, (British Museum, 1931).
[13] Gattuso, J.-P., German, D. & Frankignoulle, M. Photosynthesis and calcification at cellular, organismal and community levels in coral reefs: a review on interactions and control by carbonate chemistry. Am. Zool. 39, 160–183 (1999).
[14] Hoegh-Guldberg, O. Climate change, coral bleaching and the future of the world’s coral reefs. Tue. Freshw. Res. 50, 839-866 (1999).
[15] By degrading the protein complexes underlying, in particular, the electron transfer chains of mitochondria or chloroplasts, hyperthermia promotes the synthesis of reactive oxygen species (called ROS, for Reactive Oxygen Species) that are harmful to cells. An oxidative state has thus been demonstrated in corals subjected to hyperthermia.
[16] Collins, M. et al. in Climate Change 2013 – The Physical Science Basis (ed. Intergovernmental Panel on Climate Change) 1029-1136 (Cambridge University Press, 2013).
[17] Fabricius, K.E. et al Losers and winners in coral reefs acclimatized to elevated carbon dioxide concentrations. Nat. Air conditioning Chang. 1, 165-169 (2011).
[18] 2015 Paris Climate Conference.
The Encyclopedia of the Environment by the Association des Encyclopédies de l'Environnement et de l'Énergie (www.a3e.fr), contractually linked to the University of Grenoble Alpes and Grenoble INP, and sponsored by the French Academy of Sciences.
To cite this article: COURTIAL Lucile, ALLEMAND Denis, FURLA Paola (April 23, 2019), Corals: Ocean engineers are under threat, Encyclopedia of the Environment, Accessed April 26, 2024 [online ISSN 2555-0950] url : https://www.encyclopedie-environnement.org/en/life/corals-ocean-engineers-under-threat/.
The articles in the Encyclopedia of the Environment are made available under the terms of the Creative Commons BY-NC-SA license, which authorizes reproduction subject to: citing the source, not making commercial use of them, sharing identical initial conditions, reproducing at each reuse or distribution the mention of this Creative Commons BY-NC-SA license.