Adaptations to habitat anthropization
PDF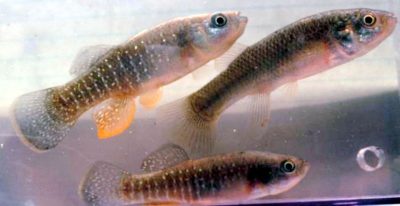
More recently, ecologists have demonstrated the genetic adaptation of earthworm (Lumbricus rubellus) populations to metal pollution in soils [2]. Similarly, although less resilient and diverse than mosquito populations, fish populations such as those of the Mummichog (Fundulus heteroclitus) have adapted to PCB contamination of their habitat [3].
However, the potential for genetic adaptation of highly abundant and fertile species to changes in their living conditions, and in particular to chemical pollution of their habitats, is not unlimited. Thus, the decline of pollinating insect species (bees and bumblebees, butterflies, beetles, etc.) observed in intensive agricultural regions for several decades is not only restricted to specialist species [4], [5]; it also concerns certain generalist species that were very abundant before the “green revolution”, such as the honeybee, whose numbers have fallen by more than 60% in 60 years [6] and whose populations do not seem to be adapting to the increasing scarcity of the flowers (messicolous plants) that they gather or to the growing toxicity of systemic pesticides (e.g. neonicotinoids).e.g. neonicotinoids) spread in the fields (and see focus 2, on The demogenetic strategy of bees).
It should also be remembered that all bird and mammal species that have inhabited cities and other anthropised environments for centuries have necessarily adapted (at least) to them behaviourally, demonstrating great capacities for innovation and/or learning by imitation. Among the generalist and mobile species that are easy to observe, let’s mention the herring gulls and laughing gulls, two ubiquitous “seabird” species that today find their pittance not only by prospecting the coastline in search of small prey and corpses (small crustaceans, molluscs, fish.), as did their ancestors, but also by following fishing boats or waiting for them in port to feed on the docks with discarded fish, by capturing earthworms that emerge in the fields during the ploughing season, by inspecting the rubbish bins of towns and villages (like cats, rats, dogs and foxes) or by feeding on organic waste in rubbish dumps.
Notes and references
Cover image. Urban herring gull. [Source: © Anne Teyssèdre]
[1] Liu N., 2015. Insecticide resistance in Mosquitoes: impacts, mechanisms and research directions. Annu. Rev. Entomol. 2015. 60:537–59.
[2] Anderson C. et al. 2017. Genetic variation in populations of the earthworm, Lumbricus rubellus, across contaminated mine sites. BMC Genetics. 18: 97-109.
[3] Nacci D.E, D. Champlin & S. Jayaraman, 2010. Adaptation of estuarine fish Fundulus heteroclitus to polyclholorinated biphenyls (PCB). Estuaries and coasts 33: 853-864.
[4] Biesmeijer J.C., Roberts S.P.M., Reemer, M. et al. 2006. Parallel declines in pollinators and insect-pollinated plants in Britain and the Netherlands. Science 313:351-354.
[5] Sanchez-Bayo F. & Kris A.G. Wyckhuys, 2019. Worldwide decline of the entomofauna: A review of its drivers. BiologicalConservation 232: 8-27.
[6] Teyssèdre A. & A. Robert, 2015. Biodiversity trends are as bad as expected. Biodiversity and Conservation 24 (3):705-706.