Sucrose or starch?
PDF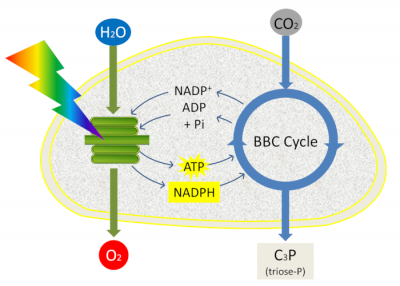
At the chloroplast level, photosynthesis consumes water, CO2 and produces oxygen and – in the case of C3 plants – phosphorylated C3 molecules (or trioses phosphate) (Figure 1). Thus the chloroplast loses a phosphate each time a triose phosphate molecule is exported to the cytoplasm. However, phosphate is required for ATP synthesis within the chloroplast. Maintaining the phosphate pool in the chloroplast is therefore essential for photosynthesis. How this can be achieved?
1. The chloroplast envelope and regulation of photosynthesis
Molecular movements must therefore take place between the chloroplasts and the cytoplasm of the cells in which they are immersed. This is where the two-membrane system (called the envelope) surrounding chloroplasts comes into play. [1]
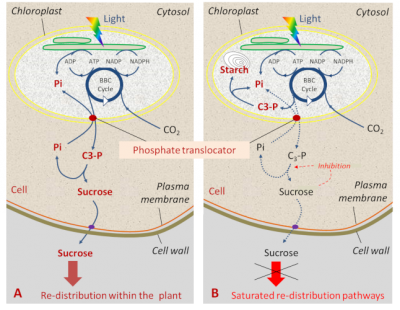
The inner envelope membrane controls the movements of many molecules between the stroma of the chloroplasts and the cytoplasm of the cell. Thus, it directs the trioses phosphate synthesized within the stroma towards the immediate (sucrose synthesis in the cytoplasm) or delayed (starch synthesis in the chloroplast) use of these molecules by the cell (Figure 2).
Like all living organisms, we are tightly dependent upon plant production and therefore on photosynthesis for our living. Thus, every carbon atom of each molecule in our body has necessarily crossed the limiting membrane of a chloroplast: once in the form of CO2 and a second one as triose-phosphate.
2. Exporting trioses phosphate for sucrose synthesis
The presence in the stroma of a large pool of phosphate (about 10 mM) allows the ATP synthase to work (see focus ATP synthesis). By exchanging a triose-phosphate molecule produced in the stroma by the Benson-Bassham-Calvin Cycle for a phosphate molecule from the cytosol, a unique protein located in the inner membrane of the envelope, the phosphate/triose phosphate translocator, maintain the phosphate pool at its optimal level.
Exported triose-phosphate molecules play a fundamental role in the economy of the cell. They will be used by glycolysis from which virtually all major metabolic pathways are derived. Very quickly their constituting carbon atoms will be found in carbohydrates, lipids, proteins and nucleic acids of the plant. This is the case for sucrose, whose synthesis releases phosphate which can return to the chloroplast. Sucrose is above all the form of transport of photosynthesis products: it moves from cell to cell to the conducting vessels (located in the veins of the leaves and stems). It is then exported to other plant territories (e.g. roots) where it will be consumed or stored, mainly in the form of starch [2]. It has been calculated that each square meter of sugar beet leaf releases about 130 mg of carbohydrates per minute into the conducting vessels.
3. Starch synthesis within chloroplasts
4. Starch as a storage form for the products of photosynthesis
- In photosynthetic leaves, starch accumulates during the day and is remobilized at night to support respiration and growth in the dark.
- In storage organs such as tubers, bulbs or seeds, starch serves as a longer-term carbon reservoir (Figure 4), which is later remobilized during seed germination, or bulb or tuber development.
In addition to its central role in plant physiology, starch is also of great economic importance. It is the second most abundant biopolymer on earth – after cellulose- and the most important carbohydrate used in food and feed. It represents the main resource of our food and the raw material for various industrial applications, such as the production of bioethanol (see Biofuels: is the future in microalgae?).
Notes and References
Cover image. [Source: Photo Eldon Newcomb © Board of Regents of the University of Wisconsin System]. Part of Figure 3
[1] Douce R. & Joyard J., 1977, Le chloroplaste. La Recherche, 8, 527-537 ; Douce R. & Joyard J., 1990, Biochemistry and function of the plastid envelope. Annu Rev Cell Biol. 6:173-216 ; Joyard J., Teyssier E., Miège C., Berny-Seigneurin D., Maréchal E., Block M.A., Dorne A.J., Rolland N., Ajlani G. & Douce R., 1998, The biochemical machinery of plastid envelope membranes, Plant Physiol. 118, 715-723.
[2] Geigenberger P. (2011) Regulation of Starch Biosynthesis in Response to a Fluctuating Environment. Plant Physiol. 155, 1566-1577.
To know more about it
- The two membranes of a chloroplast shell can be viewed interactively on the “SUN Chloroplast E-book” website
- Farineau J. & Morot-Gaudry F., 20171, La Photosynthèse, Quae, ISBN 978-2-7592-2667 2 (3rd edition)
- Pfister B. & Zeeman S.C. (2016). Formation of starch in plant cells. Cellular and molecular life sciences: CMLS, 73(14), 2781-2807. doi:10.1007/s00018-016-2250-x
- https://www.wikiwand.com/fr/Amidon