The flight of birds
PDF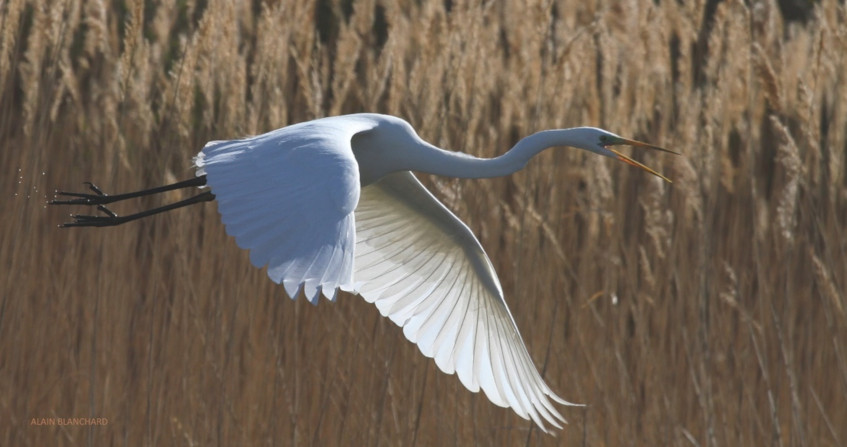
The conquest of the air by animals is a remarkable evolutionary invention that opened fantastic opportunities for many animal groups, both invertebrates and vertebrates. A multitude of organisms of all shapes and sizes, from tiny hymenoptera weighing less than 0.2 mg to enormous pterosaurs weighing up to several hundred kg, have animated or are animating the sky for more than 250 million years. By the diversity of their sizes and flight techniques, birds became masters in the evolution of flights as sophisticated as the hovering flight of the hummingbirds or the lightning swoops of a hawk pursuing its prey. Not to mention the extraordinary performances of intercontinental migrations over several thousand kilometres without stopping, even to cross high mountain ranges. A beautiful series of fossils of the ancestors of birds provide cues for understanding the essential anatomical and morphological evolution of birds, particularly that of the wings.
1. Basic principles
The diagram opposite (Figure 1) [1] illustrates the nature of the forces involved in flight. The flapping of the wing has two functions:
- the first -when the wing is lowered- consists in pushing the air downwards, which keeps the bird level thanks to the air flow which compensates the force of gravity which is all the higher as the mass is greater; this is the lift function.
- the second function – the propulsion force (also called thrust force) – propels the bird forward by producing a flow of air that slides on both sides of the wing and the body of the bird, generating a lift effect as do the wings of an airplane.
The two functions of bird wings, lift and thrust, complement each other, which explains why their shape and functioning are much more complicated than those of an aircraft wing which only provides the lift function because propulsion is provided by the engines.
The force of lift is generated by the flow of air over and under the wing, while the force of propulsion is produced when the wing is lowered during its descent, but this force is slowed down -or even cancelled- when the bird is flying on the spot, by the force of drag which is due to the resistance of the air and its friction on the body and the wing of the bird.
When the bird is cruising at constant speed and altitude, the forces of gravity and lift balance each other. For a gliding flight at constant speed, the propulsion force directed forward and the drag directed backward are balanced: the bird moves forward by inertia. If the lift is less than the weight, the bird loses altitude and if the propulsion force is less than the drag, the bird slows down. In flapping flight, each flap induces an impulse whose upward component is responsible for the lift that allows the bird to climb or descend according to its value, while the horizontal forward component, the propulsion force, can override the drag and allow the bird to accelerate.
The angle of attack of the wing is the angle formed by the chord of its aerodynamic profile and the vector of the airflow. The bird lowers or rises according to the value of this angle, exactly as do the elevators of an aircraft. The speed of flight depends on the ratio of the propulsion force to the drag force, but it also depends on the angle of attack of the wing.
A metric of major importance is the relative span or aspect ratio of the wing, which is approximated as the ratio of the length of the wing to its width (L/l). This ratio is high when the wing is long and narrow such as that of a hawk and low when the wing is short and wide such as that of a sparrowhawk. Finally, the wing loading is the ratio of the mass of the bird to the surface of its wing.
2. Profound morphological and anatomical modifications
2.1. Transformation of the skeleton of theropods at the origin of birds
2.2. An organism adapted to flight
The skeletal-muscular apparatus undertakes a spectacular evolution with an enlargement of the pelvic and pectoral girdles, an elongation of the front limbs which become the wings and the appearance of a powerful wishbone, a kind of keel on which the powerful muscles of flight are inserted. The strong antero-posterior compression of the body positions the center of gravity of the bird just under the wings. The tail is reduced to a short appendage, called “pygostyle” [3].
The internal organs also underwent adaptations that are clearly associated with flight:
- The heart, massive and powerful, is much more developed than that of a mammal of similar size (the heart of a sparrow is three times bigger than that of a mouse); it beats much faster than that of mammals, up to 1000 beats per minute in hummingbirds against 500 in mice and 70 in humans;
- The vessels are enlarged in order to transport the large quantity of oxygen necessary to the flight muscles;
- The lungs are branched into a complex system of air sacs that extend throughout the body, including the bones and pectoral muscles. The function of these sacs is to facilitate the exchange of oxygen and carbon dioxide with the bloodstream, especially when the bird flies at high altitudes where oxygen is scarce; they also function as a cooling system required by the birds’ high metabolism;
- Many other anatomical, morphological and physiological features contribute to reduce the weight of the bird: the bladder disappears, the urinary waste being evacuated with the faeces;
- The reproductive organs atrophy and resorb outside the breeding season;
Figure 4. Male superb frigatebird (Fregata magnificens). [Source photo Benjamint444, GFDL 1.2, via Wikimedia Commons] - The diet is as energetic and as light as possible, excluding poor energetic food such as leaves or grass;
- If the skeleton of the bird is very light, the musculature on the other hand is massive and powerful, totalling up to 30% of the total mass of the bird in some species. The pectoral muscles which are inserted on the wishbone are composed of a powerful pectoral muscle which brings down the wing and ensures the lift and the propulsion of the bird during the descent. A much less powerful muscle, the “supracoracoid” [4], brings up the wing.
It is probably in frigatebirds, seabirds close for boobies, that the adaptations to flight are the most remarkable. The superb frigatebird (Figure 4) weighs only 2.5 kg, half of which is for the plumage and 100 g only for the skeleton, but its wingspan reaches 2 m. This pelagic bird can neither land nor take off from a flat surface, which forces it to nest in bushes which it leaves by leaping into the air (see Focus Take-off). Having probably the lowest wing loading of all current flying birds of similar wingspan, frigatebirds fly without landing for several months, feeding on fish, squid and young sea turtles that they catch in flight. One of their specialties is stealing fish caught by other seabirds.
2.3. From the largest to the smallest

3. The different wing shapes
The shape of bird wings, which can be reduced to two metrics, the relative wingspan (L/L ratio) and wing loading, is the result of numerous compromises between the bird’s mass, its evolutionary history, its diet and its food acquisition behaviours:
- Small insectivorous forest passerines such as tits (Figure 7) and warblers benefit from having as low L/L ratio and wing loading as possible for easily gliding through the undergrowth in search of insects in tree foliage.
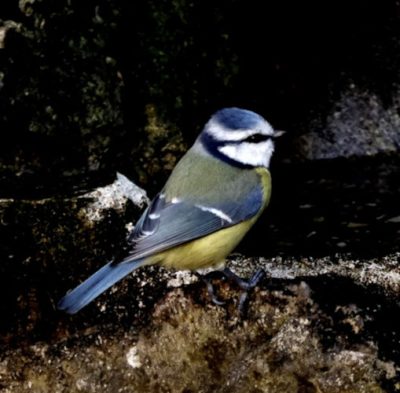
- The wing of the sparrowhawk (Figure 8A), which glides between trees while chasing a bird, is characterized by a large airfoil and a small relative wingspan that allow for good maneuverability. In contrast, the small wing area of a wing with a large relative span characterizes the fast and direct flight of a hawk (Figure 8B) which flies straight at its prey.
- Birds such as Alcidae, penguins or guillemots that “fly” in both air and water have relatively short wings with low L/L ratios, which favours them when “flying” underwater, but handicaps them when in the air.

4. The different types of theft
As a first approximation, but there are numerous variations, we can recognize two main types of flight, gliding and flapping flight, or rowing.
4.1. Gliding flight
The first is a passive flight, at least in appearance, which uses forms of energy that are external to the animal, namely the forces generated by air currents and thermal ascents produced by the topographical context of the landscapes, e.g. cliffs, mountains, inlets, coastal spaces. Gliding is characteristic of large gliders such as storks, cranes, vultures and eagles. Because they need thermal lift caused by topographical features, migration routes of these large birds are always located in regions where sea crossings are the shortest and the topographic conditions favourable to thermal lift (e.g. Gibraltar, the Bosphorus strait or the coasts of Palestine). Two reasons explain why only large species practice gliding:
- the inability of large birds to store the energy reserves required for sustained flapping flight;
- the aerodynamics of flight: large species have a much better lift/drag ratio than small ones, hence a better ratio of distance flown horizontally to distance lost vertically, which is called “glide“. The structure and properties of the wings of large gliders allow them to glide at speeds that would be far too slow to avoid stalling if their wings were as rigid as those of an airplane.
4.2. The flapping flight
The flapping flight was an immense evolutionary success that only three groups succeeded in mastering perfectly, the pterosaurs, bats and birds.
Sustained flapping flight requires a great amount of energy to compensate for the force of drag due to air resistance, to maintain the bird on its trajectory and to propel it. The propulsion is provided by the air movement of the wing during the descent. As for the upward movement of the wing, it does not generate propulsion but an important part of the lift force thanks to a twisting movement of the wing around the wrist.
- Video “How birds fly”
The frequency of the wing beats largely determines the speed of the bird, which is highly dependent on the relative span of the wing, i.e. its shape and size. The frequency varies from less than two beats per second in the great egret to more than 80 in many hummingbirds and up to 200 in the hummingbird bee (see Figure 5A), with an average of 25-27 in most small passerines.
A unique flapping flight is the hovering practiced by many birds for feeding. The sight of the kestrel doing the “Holy Ghost” over a field before swooping on its prey is a familiar image. In reality, this flight is technically quite different from another hovering flight, that practiced by hummingbirds, which are masters in this field. They are the only birds that can fly “backwards” like a helicopter. Very expensive in energy, this flight can only be practiced by very small birds when they are looking for food on a substrate on which they cannot land such as a flower.
4.3. Landing
Landing on the water is done according to the same principles, but the operation is easier because the bird can arrive much faster without risk of damage on its landing point, and then glide more or less long by raising its legs like water skis. The arrival on the water of very large birds like swans or pelicans is particularly graceful and spectacular.
5. The great adventure of migrants
The longest non-stop migratory journey recorded to date is that of a Bar-tailed godwit (Figure 13) equipped with a GPS tag, in october 2022. It completed a beaten path flight of 13,560 kilometers nonstop from Alaska to Ansons Bay in northeast Tasmania in 11 days and 1 hour — without ever touching the ground. [6]
The majority of flight altitudes are within the first 200 meters above ground or sea level, but a significant proportion of migrants fly between 1400 m and 2000 m with a distribution tail rising to 7000 m in favourable tailwinds. The highest mountain ranges such as the Himalayas are regularly flown over by geese, and the altitude record was broken by a Rüppel vulture that was sucked down by an airliner at 11,000 m. Flying at high altitude involves physiological performances that no mammal of the size of migratory birds could achieve, but it has several advantages: the atmospheric pressure decreases with altitude, which increases the lift/drag ratio. Another advantage of flying at high altitude is that it avoids most weather gusts and sudden changes in wind speed such as the Mistral wind (Mediterranean France), which can blow at more than 100 km/h but does not exceed 3000 m in altitude. Flying at such altitudes does not raise any physiological difficulties because the very particular respiratory system of birds allows them to fly at temperatures below -15°C.
6. Why not fly anymore?
6.1. Penguins: birds that “fly” underwater
not disputable, some groups have secondarily abandoned it. This is the case of penguins, where selection pressures for marine movement were stronger than those for air movement, leading to the disappearance of “air flight” in favor of “water flight” (Figure 14).
The king penguin, for example, “flies” underwater at a speed of 10-15 km/h and, like all penguins, can descend to great depths, down to 500 m.
6.2. Terrestrial giants
Even in exclusively terrestrial birds, the loss of flight can be adaptive when, for physical reasons, flight becomes impossible, especially during the take-off phase. Thus, the heaviest flying bird, the kori bustard (see Figure 5B), which weighs about 20 kilograms, is at the maximum mass possible for flight. If the selective advantage to increasing body mass continues, it is at the cost of a permanent loss of flight ability, as in the case of large ratites (emus, cassowaries, and ostriches) that may weigh up to 150 kg. It is hard to imagine what the power and muscle mass of the pterosaurs (see Figure 6) must have been because these giants that ruled throughout the Mesozoic (250 to 65 million years ago) managed to take off and fly with a mass of more than 400 kg!
6.3. Birds in insular environments
Finally, a very particular case of loss of the ability to fly is that of many birds in insular environments. The other side of the coin of active dispersal provided by flight is that it can become dangerous when one lives in a small area, ventures too far from one’s original habitat or risks of being swept away by a storm. This is evidenced by the many cases of reduction or even disappearance of the ability to fly observed repeatedly in the tree of life, both in birds and insects, as well as in the propagules of many plants.
The case of the fauna and flora of islands scattered in the immensity of the oceans is particularly interesting: while the birds of these remote islands have had to cross considerable oceanic expanses of several thousand kilometers to reach by chance the island on which they took root, they became trapped there if they manage to build a viable population. They then gradually acquire a suite of evolutionary devices whose function is to fix them to the land they have conquered [7]. Hence the acquisition of sedentary behaviours since the bird no longer go away from their island.
7. Messages to remember
- The conquest of the air by animals dates back more than 200 million years.
- An infinite number of organisms have mastered various flight techniques from tiny insects weighing less than 1 gram to such giants of the air as some pterosaurs that weighed more than 400 kg.
- Flight has favoured the conquest of an infinite number of habitats in all latitudes.
- Some groups such as penguins or many island species have abandoned the ability to fly.
- The flight techniques of birds have inspired manufacturers of flying machines through a discipline called biomimicry.
Notes & References
Cover image. Great Egret. [Source: © Alain Blanchard, reproduced with permission]
[1] Based on Burton R. (1990) Birdflight: An Illustrated Study of Birds’ Aerial Mastery. ISBN-13: 978-0816024100
[2] The synsacrum is a pneumatic bone (i.e. a hollow bone with an air-filled cavity that lightened the structure) common to birds and dinosaurs.
[3] The pygostyle is a bone (resulting from the fusion of the last vertebrae) present in the rump of birds and on which the large tail feathers (or rectrices) are attached.
[4] The supracoracoids muscles are involved for raising the flapping wing. Connected by tendons on the top of the humerus, they act as pulleys. Thus, when they contract, they pull the lowered wing upwards. They are therefore complementary to the pectorals: when the latter are contracted, the supracoracoids are relaxed, and vice versa.
[5] Alula: part of the plumage of the bird’s wing, allowing an increase in lift and reducing the risk of stalling. This would correspond to the beak of an aircraft wing. As in planes, this device allows to control the flow of the air streams which must remain laminar on the surface of the wing. The reduced speed of this type of flight makes it possible to soften the landing. See: https://fr.wikipedia.org/wiki/Alula_(bird)
[6] Marshall A. (October 27, 2022) Bird Sets Record for Nonstop 13,560km Flight. Explorer web
[7] Blondel, J. 2000. Evolution and ecology of birds on islands: trends and prospects. Life and Environment 50, 205-220. Blondel, J. & Albouy, V. 2021. Le vol chez les animaux. Quae, Versailles.
The Encyclopedia of the Environment by the Association des Encyclopédies de l'Environnement et de l'Énergie (www.a3e.fr), contractually linked to the University of Grenoble Alpes and Grenoble INP, and sponsored by the French Academy of Sciences.
To cite this article: BLONDEL Jacques (January 28, 2023), The flight of birds, Encyclopedia of the Environment, Accessed April 26, 2024 [online ISSN 2555-0950] url : https://www.encyclopedie-environnement.org/en/life/flight-birds/.
The articles in the Encyclopedia of the Environment are made available under the terms of the Creative Commons BY-NC-SA license, which authorizes reproduction subject to: citing the source, not making commercial use of them, sharing identical initial conditions, reproducing at each reuse or distribution the mention of this Creative Commons BY-NC-SA license.