Plants that live on air
PDF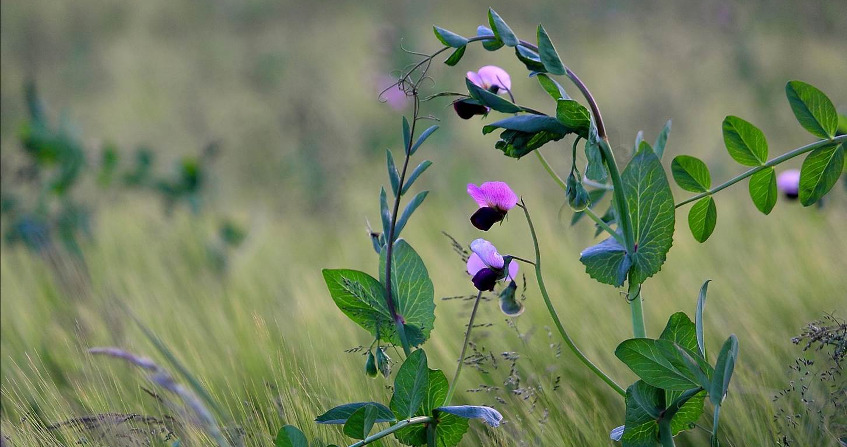
Use nitrogen from the air to synthesize its essential biomolecules? Plants achieve this feat by establishing a mutually beneficial association with soil bacteria. After a specific recognition process, the partners establish a symbiosis. Bacteria enter the root and the cortex cells divide. A specific organ is then formed, the nodule, in which atmospheric nitrogen from the air is used for the synthesis of amino acids that are then used to form proteins. This association has major economic and environmental advantages. This makes the use of nitrogen fertilizers virtually unnecessary and reduces air and groundwater pollution. These plants that live on the air of the times also have important nutritional qualities and are therefore particularly interesting.
1. Nitrogen, a plant growth limiting factor
Nitrogen (N), like carbon (C), hydrogen (H) and oxygen (O), is an essential component of life and ecosystems. It is essential to life because it is involved in the constitution of many biomolecules such as proteins, nucleic acids, nucleotides or chlorophyll. Reactive nitrogen, which can be used by plants, is present in the soil mainly in the form of nitrate (NO3–) and ammonia (NH3).
Plants obtain the nitrogen they need by absorbing it from the roots in the form of NO3– or NH3. But some species are also able to establish a relationship with marine or terrestrial bacteria that can use nitrogen from the air: this is the biological nitrogen fixation.
2. The plant – nitrogen-fixing bacteria relationship
2.1. The variety of nitrogen-fixing organisms
- Marine cyanobacteria, including filamentous cyanobacteria living in colonies (Trichodesmium, …), and unicellular cyanobacteria living either free or in symbiosis with phytoplankton (Nostoc, Anabaena,…; Figure 2). They are responsible for 40 to 50% of biological nitrogen fixation.
- Free soil bacteria, some of which are aerobic (Azomonas, Azotobacter,…) and others anaerobic (Desulfovibrio, Clostridium,…). Some are called phototrophic because they derive their energy source from light (Chromatium, Chlorobium,…), others are called chemotrophic because they use the energy of the oxidation of mineral compounds (chemo-lithotrophic: Thiobacillus, Methanococcus,…) or organic (chemo-organotrophic: Methylomonas, Azotobacter,…). They are said to be responsible for 5 to 10% of biological nitrogen fixation.
- The third category, the one of interest here, includes soil bacteria that live in symbiosis* with the root system of plants (see Symbiosis & parasitism). These are the actinomycete Frankia, which establishes symbioses with various angiosperms species (alder, sea buckthorn, Casuarinaceae; Figure 2) and Rhizobium-type bacteria that enter into symbiosis with plants of the legume family (Fabaceae) [1]. This family, which includes about 18,000 species (soybean, alfalfa, bean, lentil, groundnut, licorice, clover, wisteria, mimosa,…), is characterized by papilionaceous flowers (butterfly-shaped), a pod (fruit from the flower’s ovary) containing seeds and, for the majority of its members, the ability to use atmospheric nitrogen to produce its own nitrogen components through symbiosis with Rhizobia. [1]
2.2. How do the two partners recognize each other?
2.3. Nodules formation
Nod factors also trigger the de-differentiation (divisional entry) of cells from the root cortex, leading to the creation of a meristem* at the origin of a new organ: the nodule (Figure 4). This is gradually invaded by symbiotic bacteria, now called bacteroids. However, they are not free in the plant cells; they are surrounded by a membrane of plant origin, the peribacteroidal membrane, which will regulate exchanges between the two partners (Figure 5).
2.4. Nodule: a haven for bacteroids
Within the nodule, the oxygen concentration is strongly reduced compared to the atmospheric content. These microaerophilic* conditions allow the enzyme responsible for nitrogen fixation, bacterial nitrogenase, to be active. This enzyme is inactivated by oxygen. These conditions are allowed by the combination of two processes:
- On the one hand, a gas diffusion barrier is established in the nodule cortex thanks to layers of cells without intercellular space;
- On the other hand, infected plant cells contain a haemoprotein with a high affinity for oxygen: the leghaemoglobin.This protein, which is red in colour and has a structure comparable to animal myoglobin, provides oxygen to bacteroids at concentrations low enough not to inactivate nitrogenase.
It is important to note that when the legume grows on soil naturally rich in nitrate or ammonia, it uses the latter as a source of nitrogen. The symbiotic process is then inhibited and symbiosis does not take place.
3. Symbiotic association, a major advantage for ecosystems and agronomy
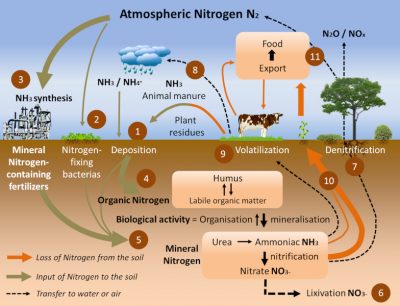
Nitrogen permanently exits and enters the soil through the nitrogen cycle (Figure 7). There are three main ways in:
- Recycling of decomposing organic matter (Step 4) [3]. Humus, derived from dead plants, crop residues and livestock manure, supplies soil and aquatic environments with organic matter (Step 1). In well oxygenated soils and aquatic environments, bacteria convert ammonia into nitrite (NO2–) and then nitrate (NO3–) during the nitrification process (Step 5).
- The spreading of fertilizers and nitrogen fertilizers (Step 5). These have been produced for more than 80 years by the Haber-Bosch chemical process [4] (Figure 8), which is used to synthesize ammonia by hydrogenation of atmospheric gaseous dinitrogen by gaseous hydrogen (H2) in the presence of a catalyst (Step 3). They now represent the main contribution of nitrogen to agriculture in industrialized countries in North and South America, Europe and Australia, and their use has increased significantly in recent decades (Figure 8).
- The biological nitrogen fixation we have described, in particular via the symbiosis between Rhizobia and legumes (Step 2). The latter, cultivated for at least 12,000 years around the Mediterranean basin, have for centuries played an essential role in the regeneration of agricultural land for nitrogen through the three-year crop rotation (a fallow year, a legume year, a cereal year). Today, biological nitrogen fixation is estimated at between 150 and 250 million tonnes per year, including about 50 million tonnes from legumes in symbiosis; by comparison, industrial production of nitrogen fertilizers by the Haber-Bosch process represents about 100 million tonnes per year [5].
- Through their roots, plants absorb nitrate and ammonia from the soil and incorporate them into amino acids, proteins and all nitrogen molecules necessary for their growth (Step 10).
- Plants are then a primary source of available nitrogen for herbivorous animals, including humans, to consume (Steps 9 & 11).
- In poorly oxygenated soils or aquatic environments, so-called “denitrifying” bacteria transform ammonia and nitrate into N2 which can be returned to the atmosphere via a denitrification process (nitrate, NO3–, to dinitrogen, N2, reduction process) (Step 7).
However, not all the nitrogen in the soil is assimilated or transformed into nitrogen. A significant part, which varies according to the nature and content of the soil in nitrogen compounds, is:
- either carried to groundwater by runoff (referred to as leaching) (Step 6),
- or volatilized into the atmosphere as ammonia or nitrogen oxides (NOx: mainly NO and NO2) (Step 8).
These losses constitute a nitrogen depletion of the soil and a source of pollution of the atmosphere and groundwater (see Nitrates in the environment).
Over the past 100 years or so, through the increase in industrial activities and the massive use of nitrogen fertilizers, human activities have significantly altered the nitrogen cycle. Today, the risk of saturation of the planet with reactive nitrogen is real and its implications for ecosystems are still poorly assessed.
4. Interest of legumes for food and the environment
4.1. A food source and a nutritional asset
In most edible legume species, the fat content of the seeds is low (1-10% of dry weight), but there are exceptions and in some species referred to as oilseeds, the seeds may contain 20% (soybean) and up to 50% (peanut) of their dry weight in fat.
While carbohydrate levels vary widely among legumes (20 to 65% of dry weight), their glycemic index* is generally low; moreover, they essentially contain a form of starch (amylose) that the body digests slowly and causes little variation in blood sugar. In addition, soluble and insoluble fibres present in seeds have a beneficial effect on digestive tract health and digestion; on the other hand, some fermentable fibres (a-galactosides) can cause risks of flatulence.
Legume seeds are also rich in vitamins (B1, B2, B3, E) and minerals (potassium, phosphorus, magnesium, zinc, iron, manganese, calcium, etc.). However, in dry seeds, the bioavailability of these compounds is reduced by the presence of anti-nutritional factors, such as protease and amylase inhibitors, or secondary metabolites such as phytates or tannins [8]. Most of these anti-nutritional factors are destroyed or inactivated by hydrothermal treatments (seed soaking and cooking), which increases the bioavailability of the various seed components.
Because of their good digestibility (80 to 90% after soaking and cooking), legume proteins can be compared to animal proteins and provide an alternative to meat consumption [2]. In terms of health and well-being, the nutritional intakes of legumes therefore have their rightful place in a varied and balanced diet. Their consumption makes it possible to better prevent cardiovascular diseases, as well as the risks of type 2 diabetes, obesity and certain cancers (colorectal, breast) [4]. A minor drawback, however, is that soybeans and especially groundnuts are highly allergenic legumes whose consumption can sometimes lead to food allergies.
4.2. A source of nitrogen and an environmental asset
Because of the symbiosis with nitrogen-fixing bacteria, legumes are therefore a natural fertilizer. They were among the first plant species domesticated in the fertile crescent more than 12,000 years ago. At the death of the plant, during the degradation of the root and aerial parts, the mineralization of organic matter releases nitrogen in forms (nitrate, ammonia, amino acids) that are easily assimilated by neighbouring plant species or subsequent crops. Today, legume cultivation accounts for about 25% of the nitrogen input to cultivated areas (about 20% in European and North American countries, and more than 50% in Asia, Africa and South America), with industrial fertilizers accounting for 63% and combustion processes for 13% [9]. The cultivation of legumes has a major environmental impact because:
- Because they require little or no fertilization, they allow a significant saving in fertilizers whose manufacturing cost is high (it takes the equivalent of 2 tons of oil to produce 1 ton of nitrogen fertilizer by the Haber-Bosch process).
- Reducing the use of fertilizers reduces groundwater pollution by reducing leaching*, which in turn reduces eutrophication*;
- They have a positive effect on the balance of greenhouse gas (GHG) emissions, including CO2, N2O or ammonia, which are mainly derived from mineral fertilizers and animal waste (symbiotic fixation produces only a small amount of N2O).
To these advantages, directly linked to the existence of symbiosis, are added those inherent to legumes, whether or not they establish a symbiosis with Rhizobium:
- They improve, by also being able to establish a mycorrhizal symbiosis, the removal of phosphorus from the soil (another factor limiting plant growth), and make it more available to other plant species.
- They have a positive impact on the soil (stabilization, limitation of runoff and erosion through the development of their root system) and a positive effect on crop rotations (reduction in fertilizer use, reduction in plant protection treatments).
- Finally, as a natural fertilizer, they have a positive effect on biodiversity by increasing the productivity of plant species, promoting pollinators (bees) and providing refuges for crop auxiliaries and macrofauna (birds, mammals).
5. Crop associations, a solution for agro-ecology
Associated crops* cereal – legume improve resource utilization compared to a single crop. This leads in particular to:
- a better grain/seed yield* than the average yield of each single crop,
- a reduction in weed biomass compared to growing legumes alone,
- and a higher protein concentration in the grains of the cereal compared to the cultivation of the cereal alone.
The advantages of the associated crop are all the more marked when the conditions are unfavourable: low yield of each crop or both grown separately or low protein content of the grain of the cereal grown alone. Associated crops are particularly beneficial when nitrogen is limiting for crops and helps to stabilize yields in organic agriculture.
The achievement of these benefits is closely linked to the proportion of legumes within the association. Thus, in grasslands, the optimal operating conditions are about 30 to 40% fodder legumes for grazed associations and 50 to 70% for mowing associations. In any case, legumes can thus be a driving force for reorienting crops towards sustainable agriculture that respects the ecological, economic and social standards that ensure that agricultural production is maintained over time.
6. Messages to remember
- Some bacteria are able to use atmospheric nitrogen for the synthesis of their biomolecules (marine cyanobacteria, soil bacteria that are free or in symbiosis with the roots of certain plants): this is the biological fixation of nitrogen.
- In the case of legumes, symbiotic bacteria penetrate the root system and cause the formation of a new organ: the nodosity. This organ is the place where atmospheric nitrogen is reduced to ammonia; it contains a protein comparable to animal myoglobin: leghaemoglobin.
- Legumes are high in protein and are an excellent complement to cereal protein for a balanced vegetable protein diet.
- The benefits of biological nitrogen fixation are multiple: economic (nitrogen fertilizer savings) and environmental (reduced air and groundwater pollution, crop associations for sustainable development).
References and notes
Cover image. [Source: © Marie-Christine Lhopital, INRA Media Library]
[1] Vegetables and legumes should not be confused. Vegetable comes from the Latin legumen which referred to plants whose fruit is a pod (pod of “legumes”). Subsequently, legumen has designated all vegetable plant species and, in its current use, a vegetable is a cultivated plant whose consumption depends on the species, leaves, roots, tubers, fruits, seeds; vegetable also refers to the consumed part of this plant. Note that in French, legumes are called “légimineuses”.
[2] Ferguson BJ, Indrasumunar A, Hayashi S, Lin M-H, Lin Y-H, Reid DE, Gresshoff PM (2010). Molecular analysis of vegetable nodule development and autoregulation. Journal of Integrative Plant Biology, 52: 61-76.
[3] The numbers in brackets refer to the nitrogen cycle diagram (Figure 7).
[4] The Haber-Bosch process makes it possible to economically fix atmospheric nitrogen in the form of ammonia, which in turn allows the synthesis of different explosives and nitrogen fertilizers. As such, from a demographic point of view, it is probably the most important industrial process ever developed during the 20th century.
[5] Erisman E, Sutton MA, Galloway J, Klimont Z, Winiwarter W (2008). How a century of ammonia synthesis changed the world. Nature Geoscience, 1: 636-639.
[6] Champ M, Magrini M-B, Simon N, Le Guillou C (2015). Les légumineuses pour l’alimentation humaines : apport nutritionnel et effets santé, usages et perspectives. In Les légumineuses pour des systèmes agricole et alimentaires durables, Schneider A & Huyghe C Coord., Editions Quae, pp 263-295. (in french)
[7] Tomé D (2012). Criteria and markers for protein quality assessment – a review. Brit. J. Nutr. 108 (S2): S222-229.
[8] Champ M, Anderson JW, Bach-Knudsen KE (2002). Supplement pulses and human health. Brit. J. Nutr, 88 (S3):S237-319.
[9] Cellier P, Schneider A, Thiébeau, Vertès F (2015). Impact environnementaux de l’introduction des légumineuses dans les systèmes de production. In Les légumineuses pour des systèmes agricoles et alimentaires durables, Schneider A & Huyghe C Coord., Editions Quae, pp 297-338. (in french)
[10] Corre-Hellou G et al. (2013). Associations céréale-légumineuse multi-services. Innovations Agronomiques 30, 41-57. (in french)
The Encyclopedia of the Environment by the Association des Encyclopédies de l'Environnement et de l'Énergie (www.a3e.fr), contractually linked to the University of Grenoble Alpes and Grenoble INP, and sponsored by the French Academy of Sciences.
To cite this article: BROUQUISSE Renaud, PUPPO Alain (August 16, 2019), Plants that live on air, Encyclopedia of the Environment, Accessed July 27, 2024 [online ISSN 2555-0950] url : https://www.encyclopedie-environnement.org/en/life/plants-that-live-on-air/.
The articles in the Encyclopedia of the Environment are made available under the terms of the Creative Commons BY-NC-SA license, which authorizes reproduction subject to: citing the source, not making commercial use of them, sharing identical initial conditions, reproducing at each reuse or distribution the mention of this Creative Commons BY-NC-SA license.