Glass: a material for eternity
PDF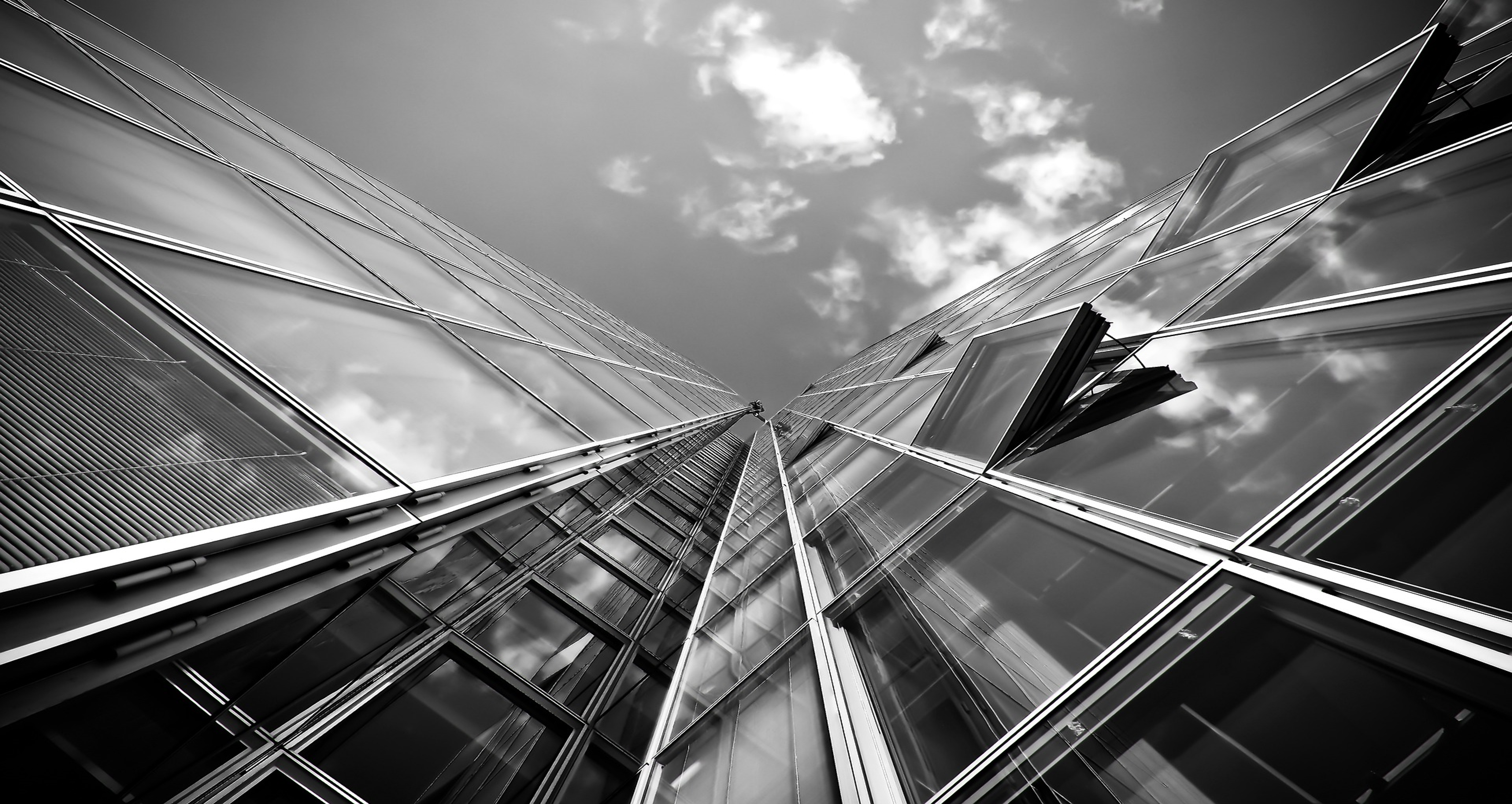
Omnipresent in our environment and now indispensable in our lives, glass is a material with extraordinary properties: transparent, tough, and durable, it can be coloured, is infinitely recyclable and therefore causes very little pollution. It is well known that glass can be made from silica, the main constituent of sand, but the means used to manufacture it and to give it the desired qualities have a long history and are still evolving. The properties and uses of each glass type depend on its chemical composition, molecular structure, and manufacturing techniques. This article is a brief extract from a recently published book [1].
1. Glass – a ubiquitous material in our environment
With around 180 million tonnes produced each year worldwide (including over 40 million tonnes in Europe), glass is one of the materials produced in very large quantities by an industry present in virtually every country in the world. The table below details production by industrial sector in Europe.
22 Mt for packaging | 800 000 t for insulating |
11 Mt flat glass | 700 000 t for specialty glass |
1,4 Mt for household goods | 160 000 t others |
The glass market, currently worth some $200 billions, is growing steadily at around 5% a year. Glass has been recycled since antiquity, thanks to its relatively simple production process. Nowadays, in Europe, this recycling is carried out using glass shavings (cullet) added to the raw materials such as sand and soda ash used to manufacture the material. The reason for the longevity of glass manufacturing and its omnipresence in our environment is due to its astonishing and unique properties.
1.1 Transparency
Glass, a material with astonishing properties, has stood the test of time, retaining its place in many aspects of our lives. Its extraordinary transparency has captivated mankind for millennia. Until the advent of plastics in the second half of the twentieth century, glass was the only material produced in large quantities at affordable prices that intrinsically possessed this precious property. Apart from rare single crystals, it was unsurpassed in this field.
This transparency is also part of our daily lives, with windows that connect us to the outside world and illuminate us, providing natural light and real aesthetics to buildings. Artists have exploited this versatility by colouring glass to create magnificent works of art, a tradition that goes back thousands of years.
1.2 Durability, hardness, uses
Glass is not only transparent, it’s also durable, as shown by the multi-century-old mirrors in the Galerie des Glaces at Versailles and the thousand-year-old stained-glass windows in Chartres Cathedral (Figure 1), which let in light while allowing nothing to be seen from the outside. Its chemical neutrality has made it an ideal tool for alchemists and chemists, resisting reactions with other substances whether gaseous, liquid, or solid.
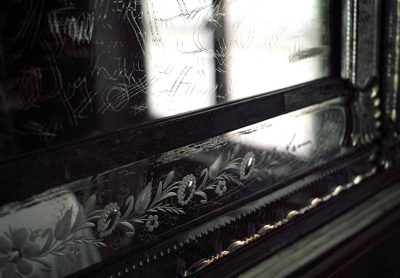
Over time, glass has been improved and strengthened to withstand high temperatures, which is essential in modern kitchens with glass-ceramic cooktops. Its mechanical strength enables it to withstand great loads in compression and stretching, an essential asset in many applications. In terms of hardness, glass is also an exceptional material, difficult to scratch except by harder and rarer substances such as diamond. Evidence of this hardness can still be seen on the mirrors of the Lapérouse restaurant in Paris (Figure 2).
Glass is also used in the dissolution of minerals, notably in nuclear waste management, where it serves as a matrix. As an electrical insulator, it has been invaluable since the earliest days of electricity, and its electronic properties continue to play an essential role in modern applications.
1.3 Viscosity
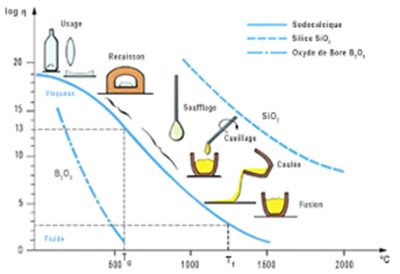
A fascinating characteristic of glass is its temperature-dependent viscosity (Figure 3), a feature that enables unique shaping thanks to various processes that rely on this property to “shape” glass. Whether it’s the historic process of glassblowing (Figure 4), still used today, or modern flat glass manufacturing processes (Float process) [2].
1.4 Suitability for recycling
2. Origin of glass
2.1 Natron, the stone from which glass is said to be made
The discovery and history of glass are fascinating tales that date back to antiquity, shrouded in a veil of mystery and legend. The romanticized story told by Pliny the Elder, in his book [4] “Natural History”, tells of Phoenician merchants who, in search of stones to support their cooking pots, used natron [5], a stone made from sodium carbonate, to raise their vessels (Figure 5) while cooking their food. Under the effect of fire, natron mixed with sand from the Belus river shore produced an unknown material, flowing in transparent streams. Thus, according to legend, was born glass.

This phenomenon is explained by the reaction of sodium with the silica (SiO2) present in the sand under the effect of heat. The sodium lowers the melting point of the silica, but this is not a simple fusion. Instead of changing abruptly from solid to liquid, the material becomes progressively softer, passing from a solid state to a kind of paste at around 800°C, then to a honey-like “liquor” at around 1000°C. At even higher temperatures, it becomes less and less viscous. Thus, the combination of sodium and silica, under the effect of heat, gave rise to glass. Although this explanation may be controversial among historians, it remains a captivating illustration of the birth of glass.
Whatever the veracity of the story, we now know that glass was already being made over a thousand years BC. At that time, there were numerous glass production centers, supplying the Mediterranean regions with “raw glass”, also known as cullet. The raw glass was then processed as close as possible to the consumer.
2.2 Fluxes other than sodium
The key to this glassmaking process is the addition of a flux, such as sodium (“soda” in glassmaking parlance), which lowers the melting point of the silica while inducing the continuous variation in viscosity characteristic of glass. Users soon noticed that sodium-silica glass was sensitive to water and deteriorated over time. The addition of calcium, mainly in the form of calcium carbonate (or “lime” in the language of glassmakers), reduced this deterioration. This mixture of silica, sodium and calcium forms the basis of soda-lime glass (solid line in Figure 3), which is one of the most common modern glass formulations, although many other compositions exist.
Sodium is not the only flux used. Potassium and boron also have this ability. Potassium, for example, has been widely used since antiquity alongside sodium, mainly from vegetable ashes. This diversity in fluxes was an asset for the glass industry in regions where access to natron was limited.
Thus, the fortuitous discovery of glass and its ability to go from solid to liquid via an intermediate pasty state were exploited by glass artisans. This made it possible to create glass objects by blowing (Figure 4), a technique found in illustrations from antiquity. This unique ability to shape glass has played an essential role in its history and use.
3. Why glass has such properties?
3.1 How to explain transparency
Transparency is an exceptional property of solid materials, especially when we look beyond synthetic plastics, which only came into being recently, in the second half of the 20th century. Apart from glass, transparent solid materials are rare. Precious or semi-precious stones, which are often coloured, are among them (Figure 6). Metals, plants and ceramics are generally opaque, except in extremely thin layers. Liquids, for their part, are frequently transparent, like water, although they can become opaque under specific conditions, for example when oil congeals.
3.2 How to explain its hardness and durability
The strength of this chemical bond is responsible for the hardness and mechanical strength of glass, as well as its durability (Figure 8). Crystals of pure silicon oxide, such as quartz, are a prime example of the strength of this chemical bond, even though they melt at high temperatures (above 1600°C).
Glass is remarkably durable and can withstand weathering and the ravages of time for thousands of years. However, despite its mechanical strength, glass is considered fragile due to its sensitivity to impact. It shatters easily when subjected to impact, which limits its applications. Glass can also shatter when subjected to rapid temperature increases. This is due to glass’s poor thermal conductivity (as an insulating material) and to thermal expansion: when glass is heated unevenly, it expands more in areas exposed to high temperature, causing mechanical stress and, eventually, material breakage.
The strong chemical bond between silicon and oxygen also makes glass resistant to most chemicals. It is commonly used in laboratories to safely contain, mix and react chemicals. The inert nature of glass also makes it ideal for preserving foodstuffs and perfumes.
Glass also can solubilize many ionic compounds, in the same way as water. Silica (SiO2) is transformed into silicates (SiO42-) by binding with positive metal ions. This is why glass can trap and dissolve a wide variety of metal ions: sodium in the classic formulation, but many other cations too. This property is the basis of the colour of many types of glass and is also exploited in applications such as the incorporation of ultimate nuclear waste, resulting from the fission of uranium in nuclear power plants, into molten glass at high temperatures for reasons of containment and stability.
4. Perspectives offered by current research
4.1 Recent innovations
Despite its long history, innovation in glass is always on the move, whether in new uses for the material or in manufacturing processes. New types of glass, such as chalcogenides [6], continue to be discovered, opening up new perspectives, particularly in the field of optical properties.
Today, specialists can create incredibly thin and flexible glass shapes, sold in rolls, and even to use glass as a base material in 3D printing to manufacture objects. Clearly, all the possibilities that glass can offer have yet to be exploited, and future discoveries will continue to broaden our horizons.
4.2 Scientific knowledge is still incomplete
However, what remains most fascinating about glass’s extraordinary history is the mystery that still surrounds its structure and properties. Despite thousands of years of use, the fundamental nature of its physical structure (amorphous structure in the solid state) is still the subject of intense scientific debate. Modern science hesitates between considering glass as an out-of-equilibrium state, comparable to a supercooled liquid, or as a new state of matter. This mystery continues to inspire the curiosity of researchers and open up new avenues of investigation. Glass therefore remains an area of constant fascination and exploration, and it is clear that new discoveries and innovations will continue to broaden our understanding of this versatile and exceptional material.
5. Messages to remember
- Glass is an exceptional material with unique properties that have led to a multitude of applications throughout history and continue to open up a host of new uses.
- Glass possesses singular properties, including transparency, hardness, durability, and the ability to solubilize various ionic compounds.
- The mixture of silica, sodium and calcium is the basis of one of the most common modern glass formulations, although many other compositions exist.
- New types of glass (chalcogenides) and new shapes (very thin, rollable or bendable glass sheets) are about to appear.
Acknowledgements
Thanks are due to all those who have contributed to the exploration and understanding of this extraordinary material. Saint-Gobain researchers, along with numerous collaborators and experts, have made an invaluable contribution to our knowledge of glass. Tribute should be paid to those who have preserved the memory and history of this material, such as Maurice Hammon, Marie de Laubier and Anne Alonzo. The proofreaders of this text, Marie Lise Roux, Pascale Fabre and Catherine Langlais, also deserve thanks for their invaluable contribution.
References and notes
[1] Didier Roux, « Le verre un matériau éternel », EDP Science, February 2024,
[2] The Float process is a revolutionary process developed by Pilkington (UK) in the 1960s which enables flat glass to be manufactured in a single step without the need for polishing and tempering. It consists of depositing a continuous ribbon of glass on molten tin. https://www.aerocontact.com/videos/93159-saint-gobain-glass-float-process
[3] The amorphous state of some solids is not specific to glass. Other materials can be prepared in amorphous form, such as certain metals which, when cooled very quickly and in thin layers, have no time to crystallize. Many organic materials are also amorphous, as are most polymers.
[4] “There is a region in Syria called Phoenicia, bordering on Judea, and containing, between the roots of Mount Carmel, a marsh called Cendevia. It is believed to give rise to the river Belus, which, after a journey of five thousand paces, flows into the sea near the colony of Ptolemaïs. Its course is slow and the water unhealthy to drink, but it is used for religious ceremonies. This deep, silty river only shows the sand it carries when the sea ebbs. Then, in fact, this sand, agitated by the waves, separates from the impurities, and cleans itself. It is thought that this contact with the sea waters influences the sand, without which it would be worthless. The coastline on which it is collected is no more than five hundred paces long, and for several centuries this was the only locality where glass was produced. It is said that nitre merchants, having disembarked there, were preparing their meal scattered along the shore; finding no stones to raise their cooking pots, they used loaves of nitre from their cargo for this purpose: this nitre, subjected to the action of fire with the sand spread on the ground, they saw transparent streams of an unknown liquor flowing, and such was the origin of glass”.
[5] Deposits of natron are nevertheless found on all continents, particularly in the soils and subsoils of salty deserts.
[6] Chalcogenide lenses are a family of lenses used mainly in optics. They are composed of chalcogen elements (sulfur, selenium, tellurium). Chalcogenide glass is opaque in the visible range and therefore often appears black to the naked eye.
.
The Encyclopedia of the Environment by the Association des Encyclopédies de l'Environnement et de l'Énergie (www.a3e.fr), contractually linked to the University of Grenoble Alpes and Grenoble INP, and sponsored by the French Academy of Sciences.
To cite this article: ROUX Didier (May 26, 2024), Glass: a material for eternity, Encyclopedia of the Environment, Accessed July 27, 2024 [online ISSN 2555-0950] url : https://www.encyclopedie-environnement.org/en/physics/glass-material-eternity/.
The articles in the Encyclopedia of the Environment are made available under the terms of the Creative Commons BY-NC-SA license, which authorizes reproduction subject to: citing the source, not making commercial use of them, sharing identical initial conditions, reproducing at each reuse or distribution the mention of this Creative Commons BY-NC-SA license.