Dew
PDF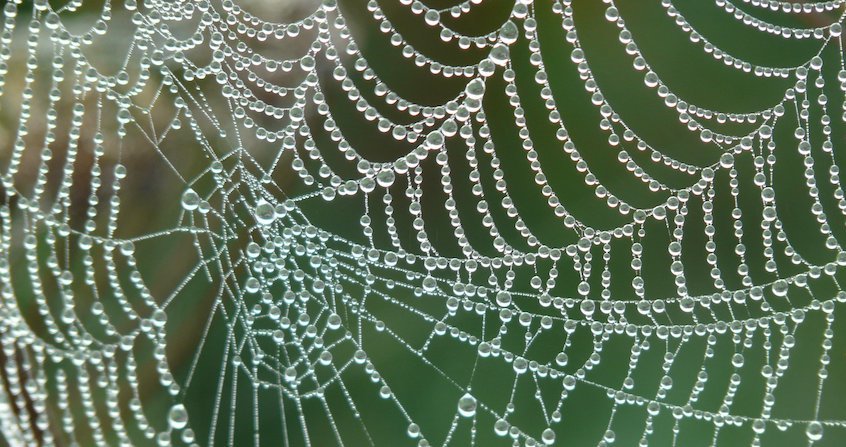
Everyone knows or has experienced dew, when the ground and plants are wet on the morning of a clear, calm night or when water runs down the walls and windows of kitchens and bathrooms. But where does dew come from ? Its origin has long been a mystery. From alchemists to scientists, many scholars have developed theories of varying degrees of rigor. It was only in the middle of the 20th century that a global interpretation of dew was developed. Dew is beneficial for plants and animals, but can humans use it as a new source of water ? How can dew be harvested ? What are the chemical and biological qualities of dew ? Is it drinkable ? All these questions are answered in this article.
1. Dew : a long history of mysteries
Everyone knows what dew is. But where does dew come from ?
Natural dew is a ubiquitous phenomenon, already mentioned in the oldest literature. For example, in the Hebrew Bible (Ecclesiastes 1:2), the famous words “All is vanity” actually correspond to the word dew in Hebrew, emphasizing the beauty and brilliance of dew but also its ephemeral nature. In Japanese culture, many haikus have been written about dew. These are just a few of the many examples of dew used in art and literature.
In contrast to natural dew, which is formed mainly outdoors, there is another dew, which is called mist, and is found on the cold walls of caves and in damp rooms, such as kitchens, bathrooms and laundry rooms. The difference comes from the origin of the cooling : the natural dew comes from a radiative exchange with the cold sky and is only effective outside, while the other “dew” comes from a cooling by contact. It is the same “dew” as the “breath patterns” that form on a glass when you breathe on it.
Men have indeed been fascinated by dew for a long time. They did not understand how water could cover the ground and plants at night when the sky was clear. An important step was taken with Leroy (1751) [1] who understood that water can dissolve in the air like sugar in water, the higher the temperature, the greater the dissolution. The cooling of hot and humid air thus inevitably leads to the extraction of liquid water, precisely at the dew point temperature. Wells (1866) [2] carried out the first complete study of dew condensation but did not explain the reason for night-time cooling. This last process was highlighted by Jamin (1879) [3] with the radiative cooling. It was only much later that Monteith (1957) [4] formalized dew formation using a complete energy balance.
1.1. Dew, a new source of water?
The first documented human use of dew water is probably the collection of dew by alchemists, noted in the book Mutus Liber (1677) [5], called the “Dumb Book” because it consists only of drawings. In Figure 1, dew is collected at night (noted by the moon) on horizontal leaves stretched on sticks. The water is then collected by pressing the leaves onto a basin. The following documented attempts concern massive dew condensers, working on temperature inertia, corresponding to a collection of mist. Such condensers are described below in section 2.3.
Radiative condensers have been the subject of several studies since the testing of massive condensers. Many areas of science and technology are indeed concerned with the process of condensation and collection of mist and dew, allowing many ideas for improvement to be experimented with: optics and atmospheric physics, radiative, conductive and convective heat exchange, hydrodynamics, chemistry, biology. Simple large planar condensers were erected (Figure 2a), more sophisticated dew factories consisting of “V” grooves (Figure 2b) were built. Many other types of radiative dew condensers (cone-shaped, origami, etc.) have been designed to increase cooling and dewdrop collection. They are presented in the book Dew Water (2018) [6].
1.2. Dew on plants
Dew condensation on plants can provide moisture and help combat periods of drought. Plants can take up water through their leaves to compensate for depleted water tables and survive drought. More complex scenarios can help plants, however. For example, the biological soil crust (Read: Lichens, surprising pioneer organisms) can retain and use dew water to increase the concentration of dissolved organic nitrogen, coupled with the fixation of atmospheric nitrogen by cyanobacteria and cyanolichens. Dew can also extend the life of seedlings under drought stress conditions.
On the other hand, dew, in addition to high relative humidity, can influence the occurrence of plant diseases, as moisture on plant surfaces promotes the development of pathogens and increases the frequency of disease in many crops. Cryptogamic diseases are observed on grass, banana and potato leaves. However, the development of these fungi can sometimes be beneficial. This is the case for the elaboration of some sweet wines. For example, the famous “noble rot” of the Sauternes vineyard is known to come from the action of the Botrytis Cinerea fungus.
2. How to collect the dew?
2.1. The dew yield
According to the previous sections, the collection surface must reach the dew point to trigger condensation. The surface area will therefore be lower than the air temperature and heat losses, increased by the wind, will be present. An energy balance can be written where, in steady state, the radiative cooling power R (in W.m-2) (see Cooling comes from the sky) counterbalances the heating by the air losses, proportional to the difference between the air temperature, Ta, and the dew point temperature, Td, and the heating by the latent heat release (Read: Pressure, Temperature and Heat), such that
Here S (in m2) is the condensation area and a (in W.m-2.K-1) is the heat transfer coefficient [7], which increases with wind speed. The condensed mass is m (kg), related to the condensed volume V (m3) by the density of the water r (kg.m3) by m = rV. The time is noted t (in s). The quantity L is the latent heat (in J.kg-1). We can easily deduce the volume of condensed water per unit area, classically expressed in liters per square meter, or mm. For the ideal case where the atmosphere has a high relative humidity , givingTa ∼ Td, the maximum efficiency, limited only by the available power (see Figure 1 in Focus Cooling comes from the sky for its value as a function of air temperature and humidity) is of the order of 1 mm per night.
2.2. The radiative dew collectors
The oldest dew condenser known to us is reported in the “Mute Book” for Alchemists (Mutus liber, 1677 [5]) where sheets are attached horizontally to sticks and then wrung out by hand (Figure 1). In dew collectors, two processes must be considered, the condensation of dew and the collection of drops. Both processes are important. According to the previous section, dew yield increases with radiative power and decreases with wind speed. In Focus 1, details on radiative cooling are given. It is shown that the cooling power is proportional to the emissivity (equal to the absorptivity) of the condensing surface – the ability of a material to emit and absorb light – and the difference in the emissivity of the atmosphere with 1. This shows that, under given conditions of atmospheric emissivity and wind speed, dew performance can be improved by (i) decreasing the emissivity of the atmosphere, (ii) increasing the emissivity of the condensing surface, (iii) decreasing the heat exchange with the surrounding air, and (iv) increasing the efficiency of dew drop collection.
The emissivity of the atmosphere (item (i)) can be reduced by considering a condenser design that utilizes only the zenith angle of the atmosphere, where the emissivity of the atmosphere is lowest (see Figure 2 of Focus 1). The emissivity of the surface (point (ii)), if not too low, is that of water and cannot be changed. However, having a substrate with low emissivity and thusabsorptivity only for the solar radiation spectrum allows for better cooling of the condenser at the end of the day and in the morning, making the dew condensation time longer.
Regarding drop collection, point (iv), passively collecting weak dew events by gravity without scraping is indeed a major challenge. There is a fundamental contradiction between having a zenith-facing surface and having a maximum surface tilt angle to facilitate gravity-based drop sliding. It has been shown [9] that a surface tilt angle between 20° and 30° gives the best results.
2.3. solid dew collectors
Soviet scientists [14], as well as their Belgian (Knapen [17]) and French (Chaptal [18]) counterparts, who had heard of the Zibold condenser, became interested again in the collection of dew water by this massive condenser technique. However, although Knapen built a fairly sophisticated condenser (Figure 5c), the efficiencies were always found to be very low because the inertia was for durations of days or weeks, not seasons. Such low efficiencies simply reflect the fact that the average temperature of massive condensers rarely falls below the dew point temperature of the air. Chaptal deconstructed his condensation pyramid “so as not to mislead future generations”. Although disappointing, the efficiencies of massive condensers could be improved by new studies taking into account the temperature of the subsoil, as for the Canadian wells where the inertia gives much longer condensation times, and by using more sophisticated technologies (Figure 5d).
2.4. Active Dew Collectors
Cooling can also be provided by an active device similar to those used in refrigerators. Power, usually electrical, must be supplied. Commercial systems exist (see for example www.candew.ca, the efficiency is of the order of 0.5 -1 kWh/L depending on atmospheric conditions. However, they are still quite expensive.
3. Can we drink the dew water ? Chemical and biological quality
Dew water is the result of the condensation of water vapor. One might think that it is as pure as distilled water. However, dew forms on a substrate outside. The dew-atmosphere and dew-substrate interactions will then give dew water its specific chemical and biological properties.
3.1. Chemical composition
Dew can interact with its substrate by partially dissolving it (e.g. when the substrate is zinc [19]). Dew also interacts with the atmosphere. The latter is characterized by gases, which can be absorbed by water, and aerosols, which settle on the substrate, acting as nucleation sites [11] for dew condensation and reacting with the condensed water. Three steps then govern the chemical composition of the dew: (i) the formation of dew on the deposited solids, (ii) the dissolution of the soluble part of these deposits by the dew water, and (iii) the absorption of gases into the dew solution.
Carbon dioxide plays a special role in the formation of liquid phase acidity because of its high and constant concentration. An important pathway in the formation of alkalinity (carbonate) is through condensation nuclei (nucleation [11] and droplet formation) as well as aerosol trapping. The ability to capture particles, e.g. CaCO3 from buildings or carbon particles from diesel cars, is very important for the chemical composition of the dew and is strong at the beginning of the condensation process and weakened at the end. The acidity of dissolved CO2, SO2 and NOx (x = 1, 2) is mainly neutralized by Mg2+, Ca2+ and NH4+; sometimes a slight alkaline character is observed in the dew samples. The dew events with the highest ionic concentration occur after long periods without rain. It should be noted that the elevated concentrations in dew water of SO2 (yielding sulfuric acid), NO (nitrous acid) and NO2 (nitric acid) are mainly ofanthropogenic origin, i.e. originating from air pollution by human activities (industry, agriculture, transport).
The absorption of very soluble gases by atmospheric water is very fast. It will therefore not be affected by the short dew formation time. In equilibrium with atmospheric CO2, the concentration of HCO3– is an exponential function of the pH value. When the pH of the solutions is above 6.35 (pKa1 of H2CO3), the [HCO3–] concentration can become significant. But the components of the atmospheric multiphase system are most likely not in equilibrium with atmosphericCO2 due to complex chemical compositions, microphysical processes and heterogeneous interactions. The concentration of HCO3– can then only be obtained by analytical estimation and not by derivation from Henry’s law [20].
The composition of dew water is thus a function of both thelong-range convected atmosphere and locally produced gases and aerosols. The source of anthropogenic and natural species can be found by various techniques, including air mass trajectories and stable isotope analyses. In general, regional urban pollution has a significant influence on dew water chemistry. In Table 1, the average composition of dew and a low mineralized spring water (Mt. Roucous) are presented for comparison; they compare relatively well.
Table 1. Chemical composition of dew measured in Bordeaux (annual average, after Beysens et al., 2006 [21]). It compares well with the Mont Roucous spring water, which has a low dissolved solids concentration.
Measurement | Dew | Mt Roucous |
pH | 5.88 | 6.0 |
Conductivity
(µS/cm) |
29 (25°C) | 25 (20°C) |
Na+, mg/l | 2.85 | 2.80 |
K+, mg/l | 0.25 | 0.40 |
Ca++, mg/l | 0.35 | 1.20 |
Mg++, mg/l | 0.35 | 0.20 |
Cl-, mg/l | 4.8 | 3.20 |
SO4–, mg/l | 2.5 | 3.30 |
NO3-, mg/l | 0.5 | 2.30 |
NO2-, mg/l | <0.01 | |
Dry residue
(180°C), mg/l |
10.3 | 19.0 |
3.2 Biological characteristics
Biological contamination of substrates comes from the deposition or droppings of insects, birds, and small mammals, decomposition of organic debris, and atmospheric deposition of suspended microorganisms, as well as from human contamination. Contamination is generally unavoidable because dew condensers are placed in an open environment. The biological effects associated with dew are different in nature depending on whether the substrate is living, such as plants, or inert.
The biological quality of dew water collected from inert substrates depends on whether the microorganisms deposited on the substrate are safe for humans. Analyses are generally performed on (i) aerobic bacteria measured in colony forming units [22] at 22 °C and (ii) at 36 °C. The first group (i) generally corresponds to harmless, plant-based microorganisms from the environment. The second group (ii) is contributed mainly by insects, bird droppings, mammals and human contamination. More specific research on human microorganisms (Enterococus, Coliforms) has also been conducted.
Contamination by microorganisms is fortunately limited by ultraviolet solar irradiation of the dew condenser surfaces [21]. Nevertheless, biological analysis of dew and rain shows that the World Health Organization limits are often exceeded. To become potable, disinfection, e.g. by chlorination, is therefore recommended.
4. Biological sterilization by condensation
The fact that condensation can occur anywhere on a substrate, even in areas of difficult access, can be used to disinfect rooms and medical instruments (e.g. endoscopes) provided that a sterilizing or antiseptic agent is added to the steam (Marcos-Martin et al., 1996 [23]). These additives are chemical vapors (e.g., ethylene oxide, formaldehyde, chlorine dioxide or hydrogen peroxide). Sterilization is indeed the result of complex chemical reactions involving alkylation or oxidation and reduction reactions, which produce free radicals such as the hydroxyl radical, one of the most powerful oxidants.
5. Take-home messages
- Dew is often mistakenly considered as a form of precipitation and confused with fog. Natural dew must also be differentiated from fog, which is the result of condensation of water on the cold walls of caves and damp rooms where the cooling comes from the thermal inertia of the wall.
- The efficiency of natural dew is mainly limited by the available cooling energy, which hardly exceeds 100 W.m-2, leading to a theoretical maximum efficiency of about 1 L.m-2 per night.
- Natural dew can make a significant contribution to the water balance and provide additional water for desert plants and animals, not only in arid and semi-arid areas, but also during dry summer seasons when drought can last for several weeks or months.
- Dew harvesting for human use has recently reached a stage of near realization due to a better understanding of the associated physics and thermodynamics, the combination of new materials and the shapes of condensers, conical, pyramidal, origami.
- The chemical properties of the dew water come from the substances (gases and aerosols) present in the atmosphere near the condenser. They can also be related to the interaction with the condensation substrate itself.
- Biological contamination of dew water comes from spores and bacteria of plant, animal and human origin. It is atmospheric in origin or comes from direct deposition by insects, birds, small mammals, humans and airborne microbes. This contamination is usually unavoidable because dew condensers are placed in an open environment. This means that dew water must be disinfected for use as a beverage.
- Sterilization by fogging. Similar to dew condensation, but indoors, sterilization of medical instruments and hospital rooms is done by condensing water with specific sterilizing elements.
Notes and references
Cover image. [Source: https://pixabay.com/ – Royalty free image]
[1] LEROY, C. (1751). Dissertation on the Elevation and Suspension of Water in the Air, and on Dew. (Dissertation on the Elevation and Suspension of Water in the Air, and on the Dew). Mémoires de l’Acad. Roy. des Sci . 481-518.
[2] WELLS, W. C. (1866). An Essay on Dew and Several Appearances Connected with it. London: Longmans, Green, Reader and Dyer.
[3] JAMIN, J. (1879). Dew, its history and role. Revue des Deux Mondes 31, 324-345.
[4] MONTEITH, J. L. (1957). Dew. Q. J. R. Meteorol. Soc. 83, 322-341.
[5] ALTUS, SAULAT J. (1677). Mutus Liber. La Rochelle: Pierre Savouret.
[6] BEYSENS, D. (2018). Dew water. Gistrup: Rivers Publisher.
[7] HEAT TRANSFER COEFFICIENT is the coefficient that relates the surface heat flux and the temperature difference at the origin of the flux.
[8] SHARAN, G., ROY, A. K., ROYON, L., MONGRUEL, A., BEYSENS, D. (2017). Dew plant for bottling water. J. Clean. Prod. 155 (1), 83-92.
[9] BEYSENS, D., MILIMOUK, I., NIKOLAYEV, V., MUSELLI, M., MARCILLAT,J.. (2003). Using radiative cooling to condense atmospheric vapour: a study to improve water yield, J of Hydrology 276 (1-4 ), 1-11.
[10] BEYSENS, D. BROGGINI, F., MILIMOUK-MELNYTCHOUK, I., OUAZZANI, J., TIXIER, N. (2013). New architectural forms to enhance dew collection. Chemical Engineering Transactions 34, 79-84.
[11] NUCLEARIZATION is the first step of formation of a new phase (here liquid) in a given phase (here vapor). It is facilitated by geometrical or chemical defects.
[12] OPUR. Available at https://www.opur.cloud/
[13] NIKOLAYEV, V., BEYSENS, D., GIODA, A., MILIMOUKA, I., KATIUSHIN, E.,MOREL, J. (1996). Water recovery from dew. J. Hydrol. 182, 19-35.
[14] MYLYMUK-MELNYCHOUK, I., BEYSENS, D. (2016). Air wells: myths and realities or Russian & Soviet works on water production from air. Saarbrücken: European University Publishing.
[15] TOUGARINOV, V.V. (1935). Condensation of atmospheric water vapour. Anonymous, 1935. Stenograph of the proceedings of the 1st Conf. on the condensation of the atmospherical water vapour (Aerial well) (1931). Moscow-Leningrad: Cuegms (in Russia). Translation (French): MYLYMUK-MELNYTCHOUK, I., BEYSENS, D. (2016). Aerial wells: myths and realities or Russian & Soviet works on water production from the air. Saarbrücken: European University Publishing.
[16] TOTCHILOV, V.I. (1938). Condensers of Feodosia and the conditions of condensation in the surroundings. Soviet Water Works and Sanitary Engineering 1 , 61-67 (in Russian).
[17] KNAPEN, M. A. (1929). Interior device of the Knapen air shaft (Interior device of the Knapen air shaft). Extract from the memoirs of the Society of Civil Engineers of France. (Bull. Jan-Feb). Imprimerie Chaix, Paris…
[18] CHAPTAL, L. (1932). The capture of atmospheric water vapour. (La capture de la vapeur d’eau atmosphérique). La Nature 60, 449-454.
[19] LEKOUCH, I., MUSELLI, M., KABBACHI, B., OUAZZANI, J., MELNYTCHOUK-MILIMOUK, I., BEYSENS, D. (2011). Dew, fog and rain as additional water sources in southwestern Morocco. Energy 36 (4), 2257-2265.
[20] HENRY’S LAW states that the amount of gas dissolved in a liquid is proportional to its partial pressure above the liquid.
[21] BEYSENS, D., OHAYON, C., MUSELLI, M., CLUS, O. (2006). Chemical and biological characteristics of dew and rain water in an urban coastal area (Bordeaux, France). Atmospheric Environment 40 (20), 3710-3723.
[22] COLONIAL FORMATION UNIT is a unit used in microbiology to quantify the number of microorganisms present in a given environment. After taking samples from the medium, cultures are made under specific conditions on a medium capable of growing the microorganisms that form colonies, which can be counted.
[23] MARCOS-MARTIN M.-A., BARDAT A., SCHMITTHAEUSLER R., BEYSENS D. (1996). Sterilization by vapour condensation. Pharm. Techn. Eur. 8, 24-32.
[24] ASP. Products. Available at https://www.asp.com/products
[25] Bioquell. Risk Reduction Solutions for Pharmaceutical, Life Sciences & Healthcare. Available at https://www.bioquell.com/life-sciences/our-technology-for-life-sciences/.
The Encyclopedia of the Environment by the Association des Encyclopédies de l'Environnement et de l'Énergie (www.a3e.fr), contractually linked to the University of Grenoble Alpes and Grenoble INP, and sponsored by the French Academy of Sciences.
To cite this article: BEYSENS Daniel (March 20, 2024), Dew, Encyclopedia of the Environment, Accessed July 27, 2024 [online ISSN 2555-0950] url : https://www.encyclopedie-environnement.org/en/air-en/dew/.
The articles in the Encyclopedia of the Environment are made available under the terms of the Creative Commons BY-NC-SA license, which authorizes reproduction subject to: citing the source, not making commercial use of them, sharing identical initial conditions, reproducing at each reuse or distribution the mention of this Creative Commons BY-NC-SA license.