Restoration of polluted soils by plants (phytoremediation)
PDF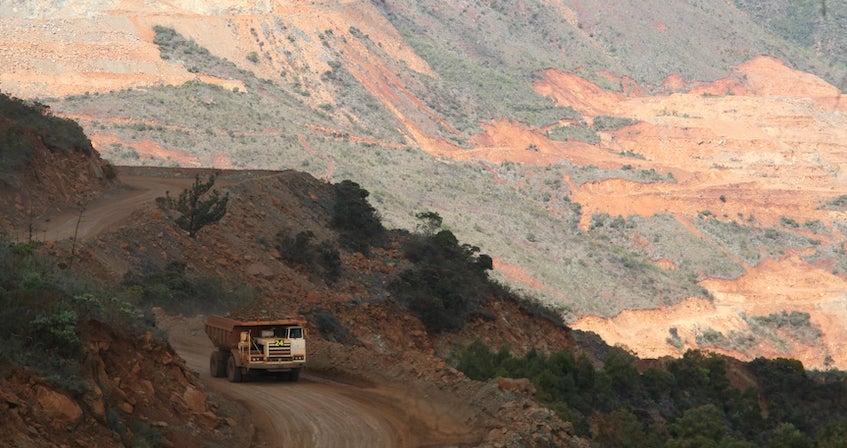
Human activities are largely responsible for soil and water pollution and more generally for global environmental damage. Each one of us has already been confronted with situations of very strong pollution or aggressions of nature, such as the impacts of biocides (herbicides, insecticides, fungicides…), hydrocarbons or metals and toxic inorganic elements (Cu, Ni, Cd, Pb, Cr, Hg, Sn, As, Tl…). Fortunately, in the long term, nature is capable of a certain resilience by adapting to certain types of pollution, in particular thanks to the activity of microorganisms and plants. This capacity of nature has long inspired mankind, especially for the treatment of their drinking water (> 3000 years). Today, nature is inspiring new approaches to integrated remediation of these polluted environments, particularly bio-inspired approaches. These approaches are part of a global ecology, which mobilizes different fields of scientific ecology (plant, microbial, molecular, evolutionary, functional, chemical…), biology, toxicology, physicochemistry and chemistry of the living. Among them, phytoremediation (a set of technologies using plants to extract, degrade or immobilize polluting compounds or elements) is one of the most promising approaches, even if it is rather intended for the resolution of environmental problems generated by trace metals, or TMEs.
1. Why do we need to remediate soils?
Countless human activities are now affecting biological dynamics and biogeochemical balances (see The biosphere, a major geological player). Strong indicators, such as climate change, biodiversity erosion, soil, river and groundwater pollution, testify to the need to develop new strategies for preserving and even rehabilitating the biosphere and associated ecosystem services. Human activities and the waste generated, whether domestic, industrial or agricultural, contribute greatly to the extent of pollution and environmental damage.
1.1. Major soil pollutants
For example, biocides [1] are chemical compounds with toxicological properties, intended to control the spread of harmful insects or rodents, algae, invasive plants or phytopathogenic fungi. Their toxicity and ecotoxicity are of concern. Residues of phytosanitary products, pharmaceutical principles, solvents, plastic residues, cosmetics… and their degradation products constitute problematic emerging pollutants. Their misuse leads to their dispersion in the air, their absorption by plants or their penetration into soils where they are then carried to aquatic environments by rainwater, contaminating rivers, groundwater and coastal areas (Read Why and how to treat urban wastewater? & Pesticides: what the past teaches us).
1.2. Significant toxicity and indirect effects
From a general point of view, their toxicity is due to their structural similarity with the essential elements, allowing them to substitute them in a competitive way, in particular in biological macromolecules such as enzymes contained in cells. Thus, for example:
- Lead is able to displace calcium in bone tissue. It is then stored in an insidious and discrete way, then released massively during a fracture, a trauma or a stress.
- In the case of copper salts, pollution is caused by frequent use (distribution pipes, electrical cables, algicides, antifungals, …) and persistence of metal species. Harmful for mammals, they are also very toxic for marine organisms [2].
Metallic pollution therefore presents real health risks: damage to the nervous, renal, pulmonary systems or bone tissue is clearly established (Read Mercury, fish and gold miners). High levels of trace metals reduce the biodiversity, density and activity of flora and fauna, even on the smallest scale. Soil fertility is altered; animals are contaminated by contact, inhalation of metallic dust, ingestion of water and food… Metallic pollutants spread through the food chain.
- Metal pollution leads to phytotoxicity of soil systems and, subsequently, to their increased erosion.
- Soil erosion leads to migration of trace metals into soil-water systems and contamination of rivers (Figure 2);
- These phenomena reduce soil fertility and lead to contamination of agricultural and food products.
1.3. Worrying consequences
The cumulative effects of climate change (droughts, intense but short rains, global warming) and the various forms of pollution mentioned above, combined with the over-consumption of water and food products, lead to fears of a shortage of vital resources. The deterioration of the quality of soils and arable land is a major concern (Figure 3). It is a major issue for world food security, which is subject to increasing constraints [2]:
- A demographic challenge: the world population is expected to reach 9 billion inhabitants in 2050 with an increased urbanization of this population (2/3 of the world population in 2050 against half today);
- Climate change/global warming: with consequences on extreme events and pressure on agricultural yields;
- Globalization of the world market for agricultural and food products, coupled with a negative evolution of eating habits;
- Increased pressure on resources, both in quantity and quality: decrease in the availability of drinking water accentuated by unequal distribution, depletion of mineral resources, decrease in the availability of arable land, changes in ecosystem services, conversion of land use, depletion of certain marine resources.
2. Phytoremediation: a natural and sustainable solution
In 2002, Isenmann conceived industrial ecology as a discipline that took nature as a model [3]. Today, this concept has been transformed into concrete courses of action that do not involve a return to an initial situation. The lack of data on the detailed characterization of the biotic and abiotic interactions of a particular ecosystem prior to industrial pollution and the physico-chemical disturbances introduced by anthropic activities, exclude the return of a natural habitat to a pre-industrial situation. It is therefore not ecological restoration in the strict sense of its definition [4]. On the other hand, the presence and the study of organisms adapted to an industrial pollution situation allow to define new integrated and bio-inspired remediation approaches. They are part of a global ecology, which mobilizes different disciplinary fields of scientific ecology (plant, microbial, molecular, evolutionary, functional, chemical…), biology, toxicology, physicochemistry and chemistry of life.
Two scenarios are possible for degraded sites: stabilization or active remediation. These remediation techniques can be broadly divided into two main sectors: bioremediation and phytoremediation.
Bioremediation is based on the already well known use of specific bacterial species adapted to the decontamination of contaminated areas [5], in particular by organic or metallic pollutants or even recently radionuclides [6], [7].
Phytoremediation is rather intended for the resolution of environmental problems generated by metallic trace elements. Due to their phytotoxicity, metal-bearing soils exert a strong selection pressure and generate particular habitats for plant species and associated micro-organisms. This results in a unique biological resource, the metallophytes [8]. These metallophytes are defined as being able to tolerate TME concentrations, and thus survive and reproduce on such sites [9].
- the phytoextraction ecocatalysis : pollutants are extracted by plants and stored in their tissues (leaves or roots);
- the phytostabilisation pollution remediation : it consists in covering the soil with plant species capable of immobilizing the pollution;
- the rhizofiltration phytoextraction: the process of phytoextraction is carried out in an aquatic environment at the level of the rhizosphere (part of the soil close to the plant roots). Phytoremediation is one of the few interesting solutions for the sustainable rehabilitation of soils degraded or contaminated by TMEs.
The most studied phytotechnologies are phytostabilization and phytoextraction [10], [11], [12], [13].
Various previous experiments have shown that phytostabilization can immobilize contaminants and contribute to the growth of vegetation in hostile areas. On the other hand, it favors the spontaneous appearance of plants that sometimes become capable of accumulating TMEs, with a risk for animals. Thus, the evolution over time of revegetated plots poses the delicate problem of controlling risks over time.
3. Some feedback from mine reclamation
In a context of rapid depletion of the world’s primary and strategic mineral resources, some of which have become critical, recycling techniques remain underdeveloped and the continued high demand for metallic elements suggests that current resources of critical metals will be depleted in the short term. These needs are leading mining operators to extend their operations and to use new extraction techniques, at the cost of significant environmental impacts: degradation of natural environments, loss of biodiversity, pollution of ecosystems, etc. [15],[16].
In order to restore (and therefore remediate) natural environments degraded or polluted by mining activities, the first research in mining reclamation consisted in developing innovative phytoremediation programs on sites and on a large scale in the main metal mining countries, and in particular in France (metropolitan France and overseas territories such as New Caledonia). The field work was most often carried out in close collaboration with the mining operators of the different countries.
Table 1. Advantages and disadvantages of phytoremediation approaches.
3.1. Phytoextraction
Phytoextraction has often been favored as one of the most promising phytotechnologies capable of bringing a social and economic dimension to rehabilitation efforts. Phytoextraction is based on the use of plants capable of extracting metallic elements from soils and concentrating them in their aerial parts. We speak of hyperaccumulative species if they are capable of containing levels of 100 mg Kg-1 of Cd or As, 1000 mg kg-1 of Co, Cu, Cr, Pb or Ni, 10,000 mg kg-1 of Mn or Zn in dry matter.
This natural technology thus contributes to the slow and progressive decrease of TME content in contaminated soils. Above all, it allows the introduction of a protective vegetation cover on the most polluted or degraded sites, which is able to limit wind and/or water erosion of rehabilitated sites.
The two illustrative examples presented below were carried out in contexts where the environmental and economic expectations of the populations concerned are very different, but where the natural adaptation phenomena of certain plants and associated micro-organisms are common.
3.2. The case of the Avinières mine in the Gard, France
In the Gard French department, the Avinières mine site is a high contamination site (500 to 800 times more Zn, Cd and Pb than the European standards). Microbial and plant ecology efforts have established a first proof of concept for large-scale remediation using phytoextraction with the strong Zn hyperaccumulators present at the site, Noccaea caerulescens and Anthyllis vulneraria (Figure 6) [17], [18].
Anthyllis vulneraria is an amazing plant species. It is one of the few legumes able to grow on highly polluted land. A. vulneraria has been identified on the mining site of Avinières (Gard).
The phenotypic, genotypic and metabolic characterization of the new bacterial species(Rhizobium metallidurans) associated with the Zn-hyperaccumulating legume, Anthyllis vulneraria, has made it possible to understand the adaptation strategies of this unusual bacterium at the genetic level, but also at the physiological and molecular levels [19], [20], [21].
The degree of local pollution is theoretically incompatible with any form of life. However, A. vulneraria is able to survive in this toxic environment. More surprisingly, it accumulates zinc to impressive and unprecedented concentrations. It is one of the strongest zinc hyperaccumulators identified to date.
- Vulneraria has become an opportunity for Zn extraction, thanks to its strong capacity to accumulate it, but also for its agronomic aptitudes, its abundant biomass, and its important root system capable of increasing the nitrogenous organic matter of the contaminated soil and thus its fertility, like any legume. It has allowed the development of a second zinc hyperaccumulator (N. caerulescens) on the mine site, which had been totally devitalized. Today, nature is slowly reclaiming its rights and other more common plant species have settled in, although it is estimated that it will take a few more decades to return to an initial ecological state.
3.3. The case of the New Caledonian open-pit mines
The success of this program has led to continued efforts to rehabilitate mining in natura in New Caledonia (Figure 3).
– New Caledonia, a global biodiversity hotspot, is home to 3,260 plant species with an endemism rate of approximately 74%. The floristic diversity of New Caledonia is closely linked to its geological history. This has led to very particular edaphic conditions (soil): a large part of the territory’s soil is rich in phytotoxic elements: Ni, Co, Cr, Mn, Fe. These very particular geochemical conditions have generated the emergence of an original flora composed of plants that have adapted to ultramafic* soils (glossary: an ultramafic rock or ultrabasic rock is a magmatic and meta-magmatic rock rich in metals (iron, magnesium, etc.) and very poor in silica (less than 45% by mass), hence their basic character).
This context is exceptional, and represents an example of remarkable adaptation of the plant world.
However, these geochemical conditions are also at the origin of intense mining, which threatens this same biodiversity. To date, more than 23,000 ha have been impacted by mining activities, leading to severe soil erosion, which pollutes the lagoon and threatens the coral reef. Since 2009, regulations have made rehabilitation mandatory (Figure 8), but only 371 ha have been revegetated because much scientific knowledge remains to be acquired, proof of the difficulty of implementing these new but complex approaches.
In this context, a new approach to mine reclamation has been developed [13].
The success of this work combines large-scale field experiments (>6 ha) and fundamental research [11], [23]. Indeed, it is still necessary to progress in the knowledge of the adaptive capacities of metallophytic plants on degraded mining sites. The objectives of remediation of degraded sites and/or sites contaminated by mining activities must clearly be deployed in the long term. The state of the sites, the reasoned and sustainable planning of the operations, the growth of the plants on bruised soils, the respect of the local biodiversity, the follow-up of the transplants, the speed of accumulation testify to this. In such a context, it is obvious that an economic valuation of the remediation is essential to support such efforts in the long term. This is “a guarantee of sustainability ” and therefore of success. A database exists, listing the metal-hyperaccumulative species associations known to date [24], some examples of which are given in the following Table 2.
Table 2. Some examples of pollutant associations with hyperaccumulative plants (as of September 2017) for phytoextraction with global records of the highest concentrations reported to date.
4. A necessary valorization of phytoextraction
The development of phytoextraction remains limited mainly by the absence of valorization of the contaminated biomass. Without a credible outlet, the aerial parts of hyperaccumulative plants are considered as contaminated waste. Moreover, the phenomenon of extraction of metallic trace elements by the root system increases the fraction of soluble elements. The development of phytoextraction is entirely linked to the valorization of the generated biomass.
The two most significant strategies for recycling plants that are hyper-accumulators of trace metals are based on two classical and independent channels:
- the sector inspired by the treatment of biomass: Bioenergy, and more particularly the wood-energy sector [25], [26];
- the process inspired by ore processing: hydrometallurgy adapted to metals of plant origin or phytoextraction (phytomining [27], [28], [29], [30], [31]).
In the case of the wood-energy sector, two immediate problems still unresolved concern the fate of combustion residues (and their possible toxicity), as well as the volatility of metallic species during combustion [16],[17]. Many questions therefore remain unanswered.
Ecocatalysis has created a paradigm shift: transition metal-rich biomass is no longer a contaminated waste product, but a natural remediation system with high added value. This biomass is therefore a natural reservoir of valuable transition metals in organic synthesis. In other words, waste has become a useful, innovative and valuable chemical object.
5. Messages to remember
- It is possible to develop phytoextraction as a technique for mining rehabilitation of degraded/contaminated soils by relying on local plant species adapted to the context of high contamination and degradation of the areas to be rehabilitated.
- Phytoextraction of contaminants is a new and promising approach; it allows to combine the introduction of pioneer and sustainable species compatible with the objectives of reclamation.
- The restoration of mining sites impacted by metallic pollution and the chemical valorisation of phytotechnologies developed by ecocatalysis make it possible to reconcile ecology, technology and economy.
- Economic activities are not translated into environmental costs, but into benefits, allowing to support the remediation efforts of polluted sites.
Notes and references
Cover image. New Caledonia, a major mining area, is a pioneer in phytoremediation [Source : © C. Grison]
[1] https://www.anses.fr/fr/content/l%C3%A9valuation-des-produits-biocides-comment-%C3%A7a-marche
[2] Allenvi GT7. http://www.allenvi.fr/, September 2, 2015
[3] Diemer A., Labrune S., L’écologie industrielle: quand l’écosystème industriel devient un vecteur du développement durable, available at: http://developpementdurable.revues.org/4121, 2 September 2015.
[4] Clewell A.F. and James Aronson, Ecological restoration, principles, values, and Structure of an Emerging Profession by IslandPress, 2nd Ed. 2013.
[5] https://www.ademe.fr/sites/default/files/assets/documents/43479_etude_adit_sols.pdf.
[6] Samakshi Verma, Arindam Kuila, Bioremediation of heavy metals by microbial process, Environ. Technol. & Innov. Volume 14, 2019,100369.
[7] Sharma B., A.K. Dangi, P. Shukla. Contemporary enzyme-based technologies for bioremediation: A review, Journal of Environ. Management, Volume 210. 2018, 10-22.
[8] Baker A.J., Ernst W.H., Van der Ent A. et al, “Metallophytes: the unique biological resource, its ecology and conservational status in Europe, central Africa and Latin America.” Ecology of Industrial Pollution, Cambridge University Press, Cambridge, pp. 7-40, 2010.
[9] Van der Ent A., Baker A.J.M., Reeves R.D. et al, “Hyperaccumulators of metal and metalloid trace elements: facts and fiction”, Plant Soil, vol. 362, pp. 319-334, 2013.
[10] Mench M., Lepp N., Bert V. et al. , “Successes and limitations of phytotechnologies at field scale: outcomes, assessment and outlook from COST Action 859”, J. Soil. Sediment, vol. 10, pp. 1039-1070, 2010.
[11] Mench M., Schwitzguebel J.P., Schroeder P. et al, “Assessment of successful experiments and limitations of phytotechnologies: contaminant uptake, detoxification and sequestration, and consequences for food safety”, Environ. Sci. Pollut. Res., vol. 16, pp. 876-900, 2009.
[12] Vangronsveld J., Herzig R., Weyens N. et al, “Phytoremediation of contaminated soils and groundwater: lessons from the field”, Environ. Sci. Pollut. Res., vol. 16, p. 765-794, 2009.
[13] Bert V., Seuntjens P., Dejonghe W. et al, “Phytoremediation as a management option for contaminated sediments in tidal marshes, flood control areas and dredged sediment landfill sites”, Environ. Sci. Pollut. Res., vol. 16, pp. 745-764, 2009.
[14] Grison C.M., Mazel M., Sellini A. Escande V., Biton J., Grison C. The leguminous species Anthyllis vulneraria as a Zn-hyperaccumulator and eco-Zn catalyst resources, Environ. Sci. Pollut. Res. 2015, 22, pp. 5667-5676.
[15] Reeves, R.D.; Baker, A.J.M. In Phytoremediation of Toxic Metals, Using Plants to Clean Up the Environment; Raskin, I., Ensley, B.D., Eds.; John Wiley: London, 2000, 232 233.
[16] Grison, C. Combining phytoextraction and ecocatalysis: a novel concept for greener chemistry, an opportunity for remediation. Environ. Sci. Pollut. Res. 2015, 22:5589-5591 and references cited.
[17] Grison C., Escande V., Biton J., Ecocatalysis: A New Integrated Approach to Scientific Ecology. ISTE Press Ltd, ELSEVIER Ltd 2015.
[18] Grison C., Combining Phytoextraction and EcoCatalysis: an Environmental, Ecological, Ethic and Economic Opportunity, Environ. Sci. Pollut. Res. 2015,22, pp 5589-5698.
[19] Grison C. M., Petit E., Dobson A., Grison C., Rhizobium metallidurans sp. nov. a symbiotic heavy-metal resistant bacterium isolated from the Anthyllis vulneraria Zn-hyperaccumulator, Int.J. Syst. Evol. Microbiology, 2015, vol. 65, pp. 1525-1530.
[20] Vidal C., Chantreuil C., Berge O. et al., “Mesorhizobium metallidurans sp nov. a metal-resistant symbiont of Anthyllis vulneraria growing on metallicolous soil in Languedoc, France”, Int. J. Syst. Evol. Micr. 2009, vol. 59, pp. 850-855.
[21] Grison C. M., Renard B.L., Grison C., A simple synthesis of 2-keto-3-deoxy-D-erythro-hexosonic acid isopropyl ester, a key sugar for the bacterial population living under metallic stress, Bioorganic Chemistry, 2014, 52C, pp. 50-55.
[22] L’Huillier L., Jaffre T., Wulff A. Mines et environnement en Nouvelle-Calédonie: les milieux sur substrats ultramafiques et leur restauration, Editions IAC, 2010.
[23] Garel C., Fonda E., Michalowicz A., Diliberto S., Boulanger C., Petit E., Legrand Y.M., Poullain C., Grison C. Structure and composition of the first biosourced Mn-rich catalysts with a unique vegetal footprint, Mat. Tod. Sust. 2019, 5, pp 100020.
[24] Reeves R.D., A.J.M. Baker, T. Jaffrée, P.D. Erskine, G. Echevarria and A. van der Ent. A global database for plants that hyperaccumulate metal and metalloid trace elements. New Phytologist (2017) 218: 407-411.
[25] Bert V., Tack K., Vialletelle F. et al. , “Prospects in biomass valorization from phytoextraction of Cd, Pb and Zn with hyperaccumulators,” Recents Progress in Process Engineering, vol. 98, p. 297, 2009.
[26] Delplanque M., Collet S., Del Gratta F. et al. 2009, “Combustion of Salix used for phytoextraction: the fate of metals and viability of the processes”, Biomass & Bioenergy, vol. 49, p. 160-170, 2013.
[27] Chaney R.L., Angle J.S., Baker A.J.M. et al., Method for phytomining of nickel, cobalt and other metals from soils, US Patent 5711784, 1998.
[28] Li Y.M., Chaney R., Brewer E. et al, “Development of a technology for commercial phytoextraction of nickel: economic and technical considerations”, Plant Soil, vol. 249, p. 107-115, 2003.
Chaney R.L., Angel J.S., Mcintosh M.S. et al. 2005, “Using hyperacumulator plants to phytoextract soil Ni and Cd”, Z Naturforsch C, vol. 60, pp. 190-198.
[30] Chaney R.L., Li Y.M., Angle J.S. et al, Phytoremediation of Toxic Elemental and Organic Pollutants, CRC Press, Boca Raton, 2000.
[31] Baker A. J.M., Echevarria G., Simmonot M.O., Morel J.L., Agromining: Farming for Metals, Extracting Unconventional Resources Using Plants. Springer International Publishing, 2018.
The Encyclopedia of the Environment by the Association des Encyclopédies de l'Environnement et de l'Énergie (www.a3e.fr), contractually linked to the University of Grenoble Alpes and Grenoble INP, and sponsored by the French Academy of Sciences.
To cite this article: GRISON Claude (January 28, 2024), Restoration of polluted soils by plants (phytoremediation), Encyclopedia of the Environment, Accessed July 27, 2024 [online ISSN 2555-0950] url : https://www.encyclopedie-environnement.org/en/soil/restoration-polluted-soils-by-plants-phytoremediation/.
The articles in the Encyclopedia of the Environment are made available under the terms of the Creative Commons BY-NC-SA license, which authorizes reproduction subject to: citing the source, not making commercial use of them, sharing identical initial conditions, reproducing at each reuse or distribution the mention of this Creative Commons BY-NC-SA license.