塔拉海洋科考队探索浮游生物的多样性
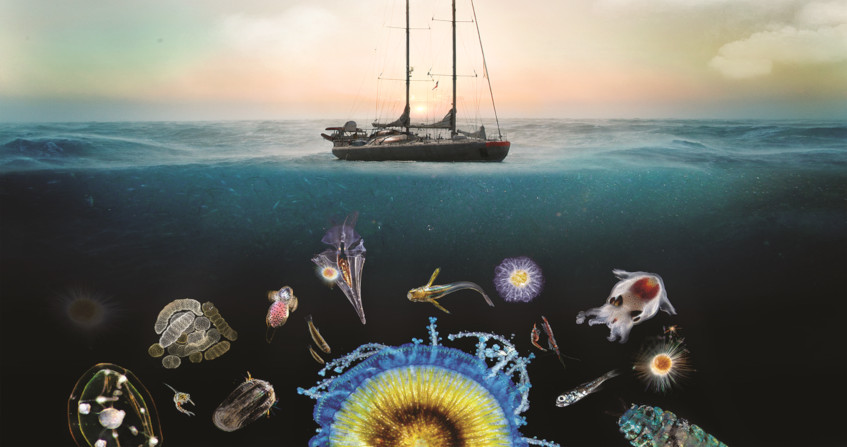
海洋覆盖了地球面积的三分之二,是30多亿年前生命诞生的地方。海洋发挥着强大的碳汇作用,吸收了人类活动排放的二氧化碳的近30%,浮游植物的光合作用主导着该过程。浮游生物是一种肉眼观测不到的生物体,在碳(地球上产生的氧气有一半来自海洋)、氮、磷、硫等元素的主要生物地球化学循环中起重要作用。然而,参与这些循环过程的微生物并不为人知。塔拉海洋科考队成立了一个国际科学联盟,为探索海洋中这类未知的生物制定了准确的采样和分析方案,生成高精度数据并进行分析。经过三年在地球海洋的航行和研究,塔拉海洋科考队的科学家们揭示了真核单细胞生物(又称原生生物)鲜为人知的多样性。近10亿的基因条形码测序从根本上改变了我们对浮游生物的种类和功能多样性的认知,明确了浮游生物对地球生态系统运作的关键意义。
1. 探索地球上最大的生态系统
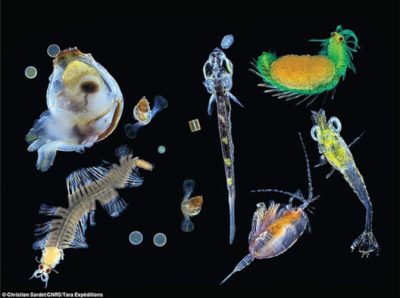
海洋是地球上最大的连续生态系统,海洋生物的生物量大部分由肉眼不可见的生物组成,即海洋微生物。其中最主要的类群是“浮游生物”。“浮游生物”(plankton)一词来自希腊语planktos,即wanderer(流浪者),指生活在水体中,不能逆流游动的生物。因此,海洋浮游生物包括原生生物(单细胞真核生物)、细菌、古生菌、病毒以及较大生物的幼虫阶段(如鱼类或甲壳类动物的幼体)(图1)。
21世纪零零年代中期,高通量DNA测序技术的出现实现了全球海洋微生物群落多样性和分布的可量化,并为大空间尺度采样提供技术支持。事实上,浮游生物的分布高度依赖于非生物因素,如光照、营养物质、湍流、温度、盐度或pH值;也受到生物因素的影响,如捕食者或共生生物。尽管浮游生物的数量存在水平和垂直空间以及季节间的差异,但该类群广泛存在于海洋中。
浮游生物对地球的重要性是多方面的:
- 浮游生物是食物链的基础,它们占地球每年初级生产力(光合作用)的50%[1]。
- 浮游生物的生产代谢对碳、氧、氮、磷和硫的主要生物地球化学循环过程起重要作用。
- 海洋吸收了近30%人类活动产生的二氧化碳(一种温室气体),这一强大碳吸收功能主要得益于浮游植物通过光合作用物捕获二氧化碳的能力(参见“人类活动对碳循环的破坏”)。
2. 塔拉海洋科考
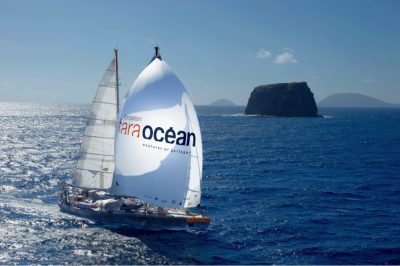
自2006年以来,塔拉号帆船上的科学家们进行了一系列科学考察活动(图2):
- 塔拉北极项目(2006-2008年):塔拉号在浮冰上漂流近两年,科学家们收集了大气、冰以及海洋数据。塔拉号在冰中停留了504天,最终到达了船只(破冰船除外)所能到达的最北位置:北纬88°32′10″ N。
- 塔拉海洋项目(2009-2013年):这是一次前所未有的大规模浮游生物研究,塔拉号行驶了14万公里,穿越地球上所有海洋。塔拉海洋极圈探险也是塔拉海洋项目的一部分,该项目绕极圈进行了为期6个月的任务,是历史上第一次在一年内跨越北极东北和西北航道。
- 塔拉地中海项目(2014年):评估了微塑料对地中海生态系统健康和功能的影响。
- 塔拉太平洋项目(2016-2018年):探索了珊瑚礁在面对全球变化时抵抗、适应和恢复的潜力。
- 塔拉微塑料项目(2019年):历经欧洲4个海岸,从9条主要河流采集样本,以追踪塑料污染的来源。
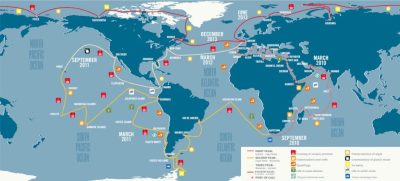
也就是说,塔拉海洋科考队探索了全球海洋表层(0-200米深)和中层(200-1000米深)水体中种类繁多的浮生物(从病毒到鱼类幼体)。
该项目从20个生物地理区的210个站点共收集了4万份海水和浮游生物样本,并探明以下问题:
- 海洋中浮游生物多样性程度如何?
- 哪些生物承担着最重要的生态功能?
- 环境参数和生物的相互作用对海洋生态系统有何影响?
为了回答这些问题,塔拉海洋科考队汇集了来自世界各地的250多名科学家。他们在这艘36米长的帆船上进行了长达三年的高度标准化取样。
塔拉海洋项目使用了许多新技术和分析工具,建立了第一个将生物地理学、生态学、遗传学和形态学结合在一起的全球数据收集工作,汇集了来自不同学科的国际团队:海洋生态学家、微生物学家、海洋学家、统计学家、生物地球化学家、计算机科学家和进化生物学家。
制定标准的取样方案旨在研究多样的海洋生态系统:上升流、生物多样性热点、低pH值或贫氧区等。为了将浮游生物的形态和基因样本置于环境背景中,研究人员总共设定了210个站点(图3),并对这些监测站进行了更精确的环境特征分析。
3. 样品收集的主要原则
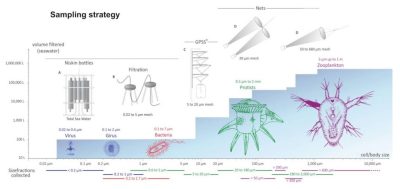
塔拉海洋科考对210个站点中的大部分(图3)都进行了三个深度的采样。
- 第一个水层是表层水(SUR),为水面以下3至7米深的水层。
- 第二个水层被称为“叶绿素最大值层”(DCM),为光合浮游生物丰度最大的水层,一般由荧光法测定叶绿素浓度来确定。浮游植物生长所必需的两种条件是光线和较冷的深层海水向上补充的营养物质,这个最大值的存在由这两个条件共同来决定。
- 第三个水层为“弱光层”,该水层位于DCM之下,是阳光能到达的最大深度,平均深度为700米。
在每一个站点,分别对不同大小范围的生物采样(图4)[2]。
在塔拉海洋科考期间收集的浮游生物大小包括六个量级,分别是:
- 病毒和巨型病毒(giant viruses,也称为giruses)(参见:“海洋病毒”);
- 原核生物(细菌和古生菌);
- 单细胞真核生物(原生生物、真菌和微藻);
- 多细胞真核生物(桡足类)。
单细胞真核生物的直径在0.8到2000微米之间。使用适当网目大小的网建立几个尺寸范围:0.8-5微米,5-20微米,20-180微米,180-2000微米。
视频1:塔拉海洋(包括下图):浮游生物采样。浮游生物过滤(通用系统模拟器)。
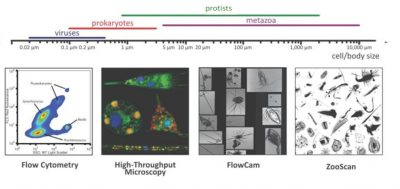
在每个站点,对不同尺寸范围的样本进行形态学分析(图5):
- 一方面,通过自动识别系统,如流式细胞摄像系统(FlowCam)[3]和浮游动物图像扫描分析系统(ZooScan)[4],能够对20微米到几厘米的生物多样性进行定量测定。
- 另一方面,通过三维共聚显微镜和透射电子显微镜可以对小型原生生物的超微结构进行分析。
4. 浮游生物的基因多样性分析
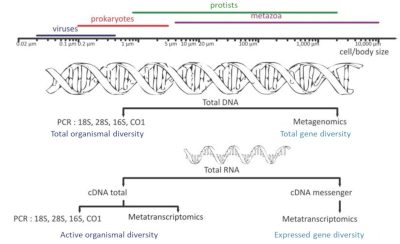
对于每个监测站点,过滤水样获得样品后,运用不同的基因分析方法(图6),让我们了解谁(何种生物)在其中,它们在做什么,它们各有何种功能或潜力:
- 通过对16S和18S rDNA(元条形码)的测序,编码核糖体RNA的基因:谁在其中?
- 元转录组学: 测定不同生物体在将基因转录成蛋白质的过程中产生的所有RNA:它们在做什么?
- 宏基因组学:环境样本中所有的基因特征:它们能做什么?
“条形码”、“转录组学”和“基因组学”等术语中添加的前缀“元”表示,这些分子分析不是对单个物种进行的,而是对来自环境样本生物群落进行的。高通量DNA测序[5][6]为定性和半定量地探索环境样本的基因多样性打开了大门。
4.1. 谁在其中?
利用不同的分子技术来探索样品的遗传多样性。
分子条形码(DNA条形码):长期以来,微生物的系统发育是基于形态学和生化特征。近年来,分子标记(条形码)被用来重建生物的进化史,其简化的技术思维是,即两个生物在进化上的距离越远,它们的基因组序列之间的差异就越大。
严格来说,DNA条形码与一个基因组的标准部分相比是一个短序列(通常为100至400个碱基对),例如:18S核糖体DNA。DNA条形码可用于识别物种,类似超市中使用的条形码,它可以确定产品(序列)和价格(物种的识别)之间的关系(参见描述生物多样性的DNA条形码)。这个序列是根据精确的标准选择的[7]:
- 种内变异性必须低:这一序列必须在同一物种的所有生物中几乎相同;
- 种间变异性必须高,才能根据它们的序列区分两个不同的物种。

用于系统发育重建最常用的DNA分子标记是编码核糖体亚基rRNA的基因,即rDNAs。在大多数真核生物中,18S rRNA存在于核糖体小亚基中,而大亚基包含3个rRNA分子(哺乳动物为5S, 5.8S, 28S,植物为25S)。编码rRNAs的基因通常聚在一起,由内部转录间隔(ITS1和ITS2)和基因间间隔(IGS)分隔(图7)[8]。
元条形码。条形码作为一种分子工具,其作用不仅是对已知物种建立高分辨率系统发育,还应用于:保护、发现物种,以及群落生态学[9],[10]。随着高通量测序的出现,分子条形码(DNA条形码)以一种元条形码的方式被广泛应用于真核生物(和原核生物!)群落生态学研究。
更正式地说,DNA元条形码是指从包含完整生物体的单一样本或包含降解DNA(来自土壤、水、粪便等)的环境样本中自动识别多个物种。用于分子元条形码研究的一个合理条形码(如18S rDNA)应该:
(i)在同一物种的个体中几乎相同的基因中的一部分,但在不同物种之间有所不同;
(ii) 适用于研究中的所有物种;
(iii) 允许在不同的水平上进行分类[6]。
样本DNA被提取后通过PCR(聚合酶链式反应)进行扩增。PCR是一种将以下状态下的DNA(或RNA)序列大量复制(增殖因子为十亿级)的技术:
- 少量的核酸(数量级低至几个皮克);
- 被称为“通用”引物的特定核苷酸引物,例如V9[12]序列,它可以特异性地扩增存在于所有个体中的特定部分DNA(扩增子,例如18S rDNA)。
因此,扩增后得到的最终PCR产物是测试样本中所有生物体的V9序列的混合物。
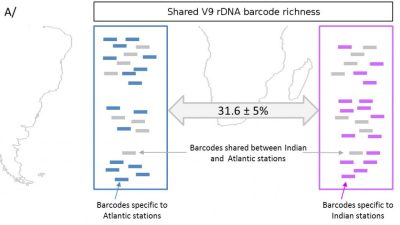
在第二步中,对扩增的副本进行测序:这样我们就得到了样本V9序列的全部多样性(图 8)[13]。具有足够相似性的序列被分组为操作分类单元(OTU)[14]。DNA序列的相似性阈值常使用97%。一个物种必须与每个OTU相匹配。
该分类的任务是通过比较样本的OTU和参考生物数据库来完成的。该方法的主要思路是查看OTU与哪个已知序列最接近,相似比例如何。相似程度会在一定程度上确定一个物种、属或科:如果一个OTU的序列与一个已知名称的物种100%相同,那么通过这个OUT即可被确定为该种类。
但有时序列不够相似,对物种的鉴定只能达到属的层面,而不能准确至种的层面。
但最大的障碍是,塔拉海洋调查所获得样品的OTU超过40%是全新的,与任何已知物种都不对应。我们只知道它们是真核生物,但几乎不可能把它们放在系统发育树中。能够发现未知的物种也令人兴奋!
4.2. 他们在做什么?
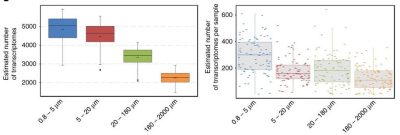
除了提供现存物种的详细目录,塔拉海洋科考队的采样还使用“元转录组学”技术。这有助于通过测序信使RNA(mRNA)来解释样本中表达的基因[15]。
为了专门研究真核生物转录组,可以只选择真核生物的mRNA(图9)[16][17]。这些mRNA在逆转录酶的作用下被逆转录:即获得的互补DNA并用于测序,然后将它们的序列与参考数据库中存在的序列进行比较,这种方法可以同时获得功能注释(这些转录本来自哪些基因,它们有什么用途?)和分类信息(这些转录本来自哪些生物种类?)。这就说明了“谁做什么”,详见Carradec等人发表的文章[17]。
4.3. 它们能做什么?
如今,基因组分析关注全基因组测序或宏基因组学,主要应用于原核生物样品[18]。全基因组分析对环境样本中的所有基因组进行测序,而不是使用特定的PCR引物(参见上文的元条形码)。
- 提取DNA后,第一步是将样本中的所有DNA片段分成非常短的片段,然后进行测序;这被称为随机序列,或称散弹枪序列。
- 随后,序列片段从重叠区域以生物信息学的方式组装,重建原始基因组。
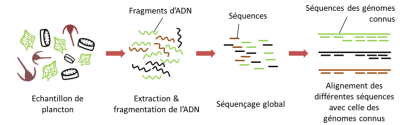
因此,宏基因组测序解释了所有未表达但存在于样本中的基因。这种类型的测序提供了关于样本中生物群落的基因潜力的信息,这就是“它们能做什么”,但不一定真的要去做。这种方法具有巨大的应用潜力但面临着许多技术的限制。
在塔拉海洋科考期间,能够用于高通量测序的DNA片段的数量刚刚超过500个碱基对。例如,如果我们考虑硅藻(一类大量存在的浮游植物)的基因组,它至少有3000万个碱基对的长度,这意味着必须对至少6万个DNA片段进行测序,才能重新构建这个完整的基因组(图10)。在实际操作中,分析从来不会对单个个体进行,而是对不同个体的混合DNA片段进行。
在实际操作中,我们的测序不可能限制于在这6万个片段中随机进行,因为一旦这么做,硅藻基因组的某些部分将被多次重复测序,而其它部分则根本没有被测序,因此重建的基因组将会有“缺陷”。DNA的重叠片段也需要重建序列顺序。
为了解决这个问题,测序过程不仅对单个基因组(60,000个片段)进行,而是对20个基因组(1,200,000个片段!)进行。
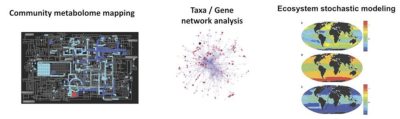
在塔拉海洋考察中,对水体内成百上千的物种的基因组进行测序似乎是一项艰巨的任务。但完成该任务具有重要意义:大多数被识别的基因(>50%)与现有数据库中已有的基因没有同源性,这引发了很多思考。对基因序列进行组装[19]和注释[20]的挑战是巨大的,但每年都在进步。
结合定量图像,使用这些高通量测序方法不仅可以理解基因的结构、位置和功能,而且最重要的是,可以探索浮游生物的多样性。利用基于生物信息学的强大方法,这些分析提供了生物之间的进化、代谢和相互作用的数据(参见:海洋生物碳泵),并实现了群落代谢组、基因和生物网络以及物种空间分布模型的重建(图11)。
5. 惊人的多样性:浮游原生生物
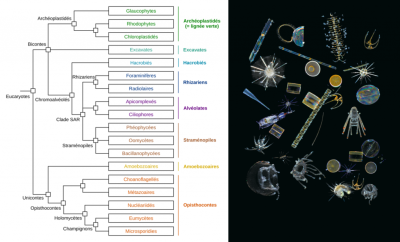
经过三年的航行和对海洋中受光照上层水体至底层的研究,塔拉海洋项目的研究人员发现了真核单细胞生物(也称为原生生物)巨大的多样性(图12)[21]。对近10亿个基因条形码的测序表明,原生生物的多样性远超过细菌或动物,它们中的大多数种类鲜为人知,包括了寄生虫、共生体和捕食者等所有群体。这些结果从根本上改变了我们对世界浮游生物的种类和功能多样性的看法,浮游生物是我们生物圈运作的关键生态系统。
- 在“海洋之家(Maison des Océans)”上展示塔拉海洋的科考成果
在这项研究中,研究人员仅从46个采样点就破译和分析了近10亿个核糖体DNA序列。这些序列被用作真核生物多样性的标志(参见:“什么是生物多样性?”以及“生物多样性不是奢侈品,而是必需品”),标记从最小的单细胞生物(小于1微米)到几毫米大小的浮游动物[22]。
生成的大量基因条形码首先使我们能够分析透光水层中几乎所有的真核浮游生物特征,鉴定出15万种遗传类型的真核浮游生物,与迄今为止所描述的1.1万种生物相比,这是一种未曾预料到的多样性。所列出的绝大多数基因类型在目前的基因数据库中似乎没有相似的参照物,说明这些生物大多是未被记录和不可培养的。其中三分之一的基因多样性与当今已知的任何主要真核生物系无关联。
在现有的真核生物谱系中可分类的的基因类型中,大多数种类与单细胞生物(或原生生物)相对应,其生物类群多样性惊人,包括了寄生虫、共生物种和捕食者等。光合作用生物的多样性少得多,体型小得多,生物量也少得多。
到目前为止,有100多篇论文是直接从塔拉海洋科考队的数据分析中得出的,包括五篇原创发表于2015年《科学》杂志“塔拉海洋”特刊的文章(仅使用“塔拉海洋”一词查询2009-2019年ISI Web of Science数据库;2020年10月2日)。
6. 要记住的信息
- 经过三年的环球航行,塔拉海洋科考队的研究人员对浮游生物进行了详尽的研究,从而能够全面了解全球浮游生物生态系统。
- 这个项目已经从4万多个海水样本中发现了1.5亿个海洋基因、10万个基因定义物种以及决定浮游生物群落的分布的因素。还揭示了原生生物(单细胞真核生物)未知的多样性。
- 在领先期刊上发表的100多篇科学论文来自于对塔拉海洋科考队所获数据的直接分析结果。所有科学家都可以在公共数据库中免费获得这些数据。
- 在开展这些研究活动的同时,塔拉海洋项目还旨在提高公众对气候变化相关问题的认识,其方法是通过开展大量工作坊、船上访问、提供教育工具等,这些途径都经由科学家验证,在其网站上可以获取。
- 由此产生的科学也被用于气候治理:作为联合国的特别观察员,塔拉海洋科考队动员了最高层的政治决策者。
- 塔拉海洋最初的科学组织: EMBL科学主任埃里克·卡森提(Eric Karsenti)、塔拉科考总监艾蒂安·布尔吉斯(Etienne Bourgois)、塔拉科考秘书长罗曼·特鲁布雷(Romain Troublé)、EMBL的斯蒂芬妮·坎德尔斯·刘易斯(Stephanie Kandels Lewis)和DVIP 咨询公司业务经理迪迪埃·韦拉尤东(Didier Velayoudon)。
- 塔拉海洋科学协调员: 这一跨学科的国际小组制定了采样战略和总体计划,确定了总体考察和项目目标,领导了考察工作,并在过去十年中对大部分结果进行了分析。见参考文献 [23]。
参考资料及说明
封面照片:[G.Bounaud; C.Sardet; La Niak;塔拉海洋基金会]
[1] Field CB. 1998. Primary production of the biosphere: integrating terrestrial and oceanic components, Science 281, 237-240.
[2] 用多聚甲醛保存的水样用于高分辨率显微镜。
[3] FlowCam https://www.embrc-france.fr/fr/prestation/flowcam
[4] ZooScan https://www.embrc-france.fr/fr/prestation/zooscan
[5] 在塔拉海洋科考期间收集的浮游生物的DNA和RNA在国家基因测序中心进行了分析。该中心创建于1996年,用于参与人类基因组计划并在法国开发基因组计划,而后研究环境基因组学和宏基因组学。
[6] Dardel F. & Képès F., 2002, Bioinformatics: genomics and post-genomics, Éditions de l’École Polytechnique 153-180 p. (ISBN 978-2-7302-0927-4, 在线阅读).
[7] Valentini A, Pompanon F, Taberlet P. 2009. DNA barcoding for ecologists, Trends in Ecology & Evolution, 24, 110-117.
[8] Zagoskin M, Lazareva V, Grishanin A & Mukha D. 2014. Phylogenetic information content of Copepoda ribosomal DNA repeat units: ITS1 and ITS2 impact. BioMed Research International, 926342. https://doi.org/10.1155/2014/926342
[9] Kress WJ, Erickson DL, Uriarte M & Garcı C. 2015. DNA barcodes for ecology, evolution, and conservation. Trends in Ecology & Evolution, 30, 25-35.
[10] Bucklin A, Lindeque PK, Rodriguez-Ezpeleta N, Albaina A & Lehtiniemi M. 2016. Metabarcoding of marine zooplankton: prospects, progress and pitfalls. Journal of Plankton Research, 38, 393-400.
[11] Taberlet P, Coissac E, Pompanon F, Brochmann C & Willerslev E. 2012. Towards next generation biodiversity assessment using DNA metabarcoding, Molecular Ecology, 21, 2045-2050.
[12] 通过比较不同生物体之间已知的18S rDNA序列,确定了一个名为V9的区域。它有130个碱基对长,它的末端在所有已知的生物中都高度保守。因此,在PCR过程中,该序列的末端可以作为引物的锚点。
[13] Villar E. et al (2015) Environmental characteristics of Agulhas rings affect interocean plankton transport. Science DOI:10.1126/science.1261447
[14] Blaxter M, Mann J, Chapman T, Thomas F, Whitton C., Floyd R & Eyualem A. 2005. Defining operational taxonomic units using DNA barcode data. Philosophical Transactions of the Royal Society B: Biological Sciences, 360, 1935-1943.
[15] 信使RNA或mRNA是对应于一个或多个基因的部分DNA的短暂拷贝。信使RNA被细胞用作蛋白质合成的媒介。在细胞中,mRNA群与表达的基因相对应,然后被翻译成蛋白质。
[16] 这些是所谓的多腺苷化mRNA:它们存在于由一系列腺苷组成的尾部的3 ‘部分,而原核生物中没有(见参考文献[6])。
[17] Carradec Q. et al. 2018. A global ocean atlas of eukaryotic genes. Nature Communications 9, 1038.
[18] 萨查·舒茨(Sacha Schutz)元基因组学简介
[19] 组装包括对长序列的DNA或RNA片段进行比对和/或融合,以便使用生物信息学工具重建原始序列。组装的问题可以比作将一本书从之前被撕成小块的几份中重新构建文本的问题。
[20] 基因组注释是对构成原始信息的核苷酸序列进行分析,提取生物信息。它一方面可以定位基因和编码区域,另一方面可以识别或预测它们的生物学功能(这是功能注释)。这两个步骤最初是基于使用复杂的算法工具,其发展是生物信息学的一个领域。
[21] Lecointre G & Le Guyader H. 2016. Classification phylogénétique du vivant – Tome 1, Éditions Belin, Collection Nature, 584 pp. (法语)
[22] by Vargas C, Audic S, Henry N, Decelle J, Mahe F, Logares R, Lara E, Berney C, Le Bescot N, Probert I, Carmichael M, Poulain J, Romac S, Colin S, Aury JM, Bittner L, Chaffron S, Dunthorn M, Engelen S, Flegontova O, Guidi L, Horak A, Jaillon O, Lima-Mendez G, Lukes J, Malviya S, Morard R, Mulot M, Scalco E, Siano R, Vincent F, Zingone A, Dimier C, Picheral M, Searson S, Kandels-Lewis S, Acinas SG, Bork P, Bowler C, Gorsky G, Grimsley N, Hingamp P, Iudicone D, Not F, Ogata H, Pesant S, Raes J, Sieracki ME, Speich S, Stemmann L, Sunagawa S, Weissenbach J, Wincker P & Karsenti E. 2015. Eukaryotic plankton diversity in the sunlit ocean. Science, 348, 1261605.
[23] 塔拉海洋的科学协调员包括:
Silvia Acinas, Department of Marine Biology and Oceanography, Institut de Ciències del Mar (CSIC), Barcelona, Catalonia, Spain.
Peer Bork, Structural and Computational Biology, European Molecular Biology Laboratory, Meyerhofstr. 1, 69117 Heidelberg,Germany.
Emmanuel Boss, School of Marine Sciences, University of Maine, Orono, Maine 04469, USA.
Chris Bowler, PSL Research University, Institut de Biologie de l’Ecole Normale Supérieure (IBENS), CNRS UMR 8197, INSERM U1024, 46 rue d’Ulm, F-75005 Paris, France.
Colomban de Vargas, CNRS, UMR 7144, EPEP & Sorbonne Universités, UPMC Université Paris 06, Station Biologique de Roscoff, 29680 Roscoff, France.
Mick Follows, Department of Earth, Atmospheric and Planetary Sciences, Massachusetts Institute of Technology, Cambridge, MA, USA.
Gaby Gorsky, Sorbonne, UPMC Université Paris 06, CNRS, Laboratoire d’oceanographie de Villefranche (LOV), Observatoire Océanologique, 06230 Villefranche-sur-Mer, France.
Nigel Grimsley, CNRS, UMR 7232, BIOM, Avenue du Fontaulé, 66650 Banyuls-sur-Mer, France.
Pascal Hingamp, Aix Marseille Univ, Université de Toulon, CNRS, IRD, MIO, Marseille, France.
Daniele Iudicone, Stazione Zoologica Anton Dohrn, Villa Comunale, 80121 Naples, Italy.
Olivier Jaillon, Genoscope, Institut de biologie François Jacob, Commissariat à l’Energie Atomique (CEA), CNRS, Université Evry, Université Paris-Saclay, Evry, France
Lee Karp-Boss, School of Marine Sciences, University of Maine, Orono, Maine 04469, USA.
Uros Krkic, Cell Biology and Biophysics, European Molecular Biology Laboratory, Meyerhofstrasse 1, 69117 Heidelberg, Germany.
Fabrice Not, CNRS, UMR 7144, Sorbonne Universités, UPMC Université Paris 06, Station Biologique de Roscoff, 29680 Roscoff, France.
Hiroyuki Ogata, Institute for Chemical Research, Kyoto University, Gokasho, Uji, Kyoto, 611-001, Japan.
Stephane Pesant, MARUM, Center for Marine Environmental Sciences, University of Bremen, Bremen, Germany.
Jeroen Raes, Department of Microbiology and Immunology, Rega Institute, KU Leuven, Herestraat 49, 3000 Leuven, Belgium.
Emmanuel Reynaud, Earth Institute, University College Dublin, Dublin, Ireland.
Christian Sardet, Sorbonne Université UPMC Université Paris 06, CNRS, Laboratoire d’oceanographie de Villefranche (LOV), Observatoire Océanologique, 06230 Villefranche-sur-Mer, France.
Mike Sieracki, National Science Foundation, Arlington, VA 22230, USA.
Sabrina Speich, Laboratoire de Physique des Océans, UBO-IUEM, Place Copernic, 29820 Plouzané, France.
Lars Stemmann, Sorbonne, UPMC Université Paris 06, CNRS, Laboratoire d’oceanographie de Villefranche (LOV), Observatoire Océanologique, 06230 Villefranche-sur-Mer, France.
Matthew Sullivan, Department of Microbiology, The Ohio State University, Columbus, OH 43214, USA.
Shini Sunagawa, Structural and Computational Biology, European Molecular Biology Laboratory, Meyerhofstr. 1, 69117 Heidelberg, Germany.
Jean Weissenbach, Genoscope, Institut de biologie François Jacob, Commissariat à l’Energie Atomique (CEA), CNRS, Université Evry, Université Paris-Saclay, Evry, France
Patrick Wincker, Genoscope, Institut de biologie François Jacob, Commissariat à l’Energie Atomique (CEA), CNRS, Université Evry, Université Paris-Saclay, Evry, France
环境百科全书由环境和能源百科全书协会出版 (www.a3e.fr),该协会与格勒诺布尔阿尔卑斯大学和格勒诺布尔INP有合同关系,并由法国科学院赞助。
引用这篇文章: VINCENT Flora, BOWLER Chris (2024年3月11日), 塔拉海洋科考队探索浮游生物的多样性, 环境百科全书,咨询于 2024年4月26日 [在线ISSN 2555-0950]网址: https://www.encyclopedie-environnement.org/zh/vivant-zh/the-tara-oceans-expedition-explores-the-diversity-of-plankton/.
环境百科全书中的文章是根据知识共享BY-NC-SA许可条款提供的,该许可授权复制的条件是:引用来源,不作商业使用,共享相同的初始条件,并且在每次重复使用或分发时复制知识共享BY-NC-SA许可声明。