What is biodiversity?
PDF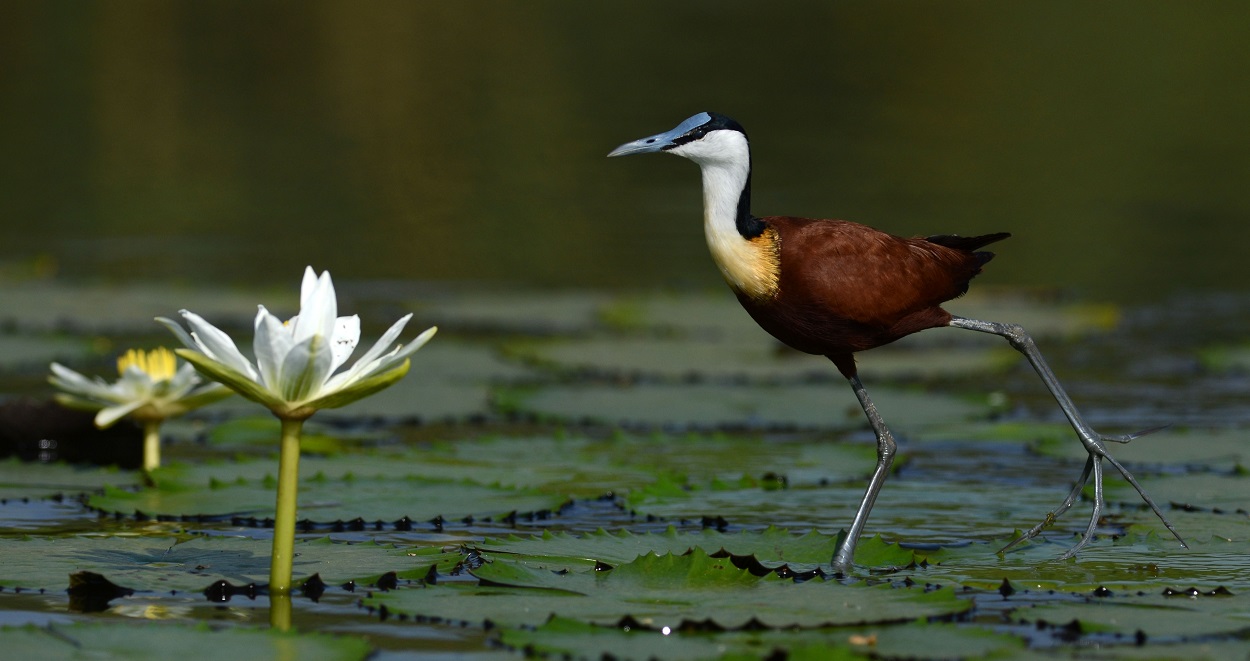
Biodiversity concerns all living organisms, their interactions with each other and with their environment. All levels of life are concerned: from the gene to the individual, then to the species and their populations, to species associations within ecosystems. The tree of life illustrates the biodiversity of species and reflects the relationship between them and makes it possible to understand their evolutionary history. Broadly speaking, an ecosystem is therefore characterized by interactions, flows of matter and energy between each of the components of the ecosystem and a dynamic balance over time, between sustainability and evolution, resilience and resistance to disturbances affecting it.
“What is essential is invisible to the eye,” the little prince repeated, so that he would be sure to remember.
The Little Prince, Antoine de Saint-Exupéry
1. Definition
The concept of biodiversity is a recent one. In 1984, Edward O. Wilson published “Biological diversity“, which puts forward for the first time the idea of biological diversity. But this new concept only really took off with the signing of the Convention on Biological Diversity at the 1992 Rio Earth Summit. In its Article 2, this Convention defines biodiversity as “the variability of living organisms from all sources, including, inter alia, terrestrial, marine and other aquatic ecosystems and the ecological complexes of which they are part; this includes diversity within species, between species and between species and as well as that of ecosystemsAll formed by an association of living beings (or biocenosis) and its biological, geological, edaphic (soil), hydrological, climatic and other environments (biotope). An ecosystem is characterized by interactions between living species and their surrounding environment, matter and energy flows between each of the ecosystem components that allow their life and a dynamic balance over time between sustainability and evolution.“. Ecologist Robert Barbault sums up this definition as follows: it is “life, in its diversity“.
Biodiversity therefore concerns all living organisms, the interactions they have with each other and with the environment in which they live. All levels of life are concerned: from the gene to the individual, then to the species, in close interaction with the environments in which they are found and with the species that surround them, and in particular ecosystems. Biodiversity must also be considered on the scale of the planet’s history: life appeared on Earth about 3.8 billion years ago (see the series of articles on the Emergence of Life) and the current state of biodiversity is therefore the result of a very long evolutionary process.
However, the sustainability of the biological and genetic resources of living organisms and their living environments are social, economic, legal and political constructs whose issues relate to the interactions of human societies with the biosphere as a whole: access to resources, uses made of them, benefits derived from them, sharing, management, sustainability, etc. Finally, biodiversity is an ethical issue because it raises the question of the right to life of species, which can be considered as imprescriptible, as defended by several very active philosophical currents such as environmental ethics (see Focus on Environmental Ethics). Biodiversity is therefore also part of the Human and Social Sciences, as described in the article Biodiversity is not a luxury, but a necessity by Jacques Blondel.
2. Species diversity
This level of understanding of biodiversity is, a priori, the most intuitive as it distinguishes between species. We easily differentiate between various animals or plants that surround us: we know what a lily, a spider, a penguin or a leopard are (Figure 1). But the very definition of the case is not so simple. For the zoologist and systematistBiologist who studies Systematics, the science of taxon classification. He uses a system to count them and, above all, to classify them by organizing them in a certain order, on the basis of logical principles. Guillaume Lecointre: “In nature, there are no species. There are only reproductive barriers. We create species from a theoretical model” [1]. To put it in a nutshell, a species can be said to be a group of living beings having a similar appearance, fertile among themselves and generating, under natural conditions, viable and fertile offspring. But this definition does not really apply to microorganisms, such as bacteria: invisible to the naked eye, they are very difficult to distinguish on the basis of simply morphological criteria.
The description of existing or extinct species is essential to make an inventory and organize them among themselves. Initially, each species was known by various common names, depending on the regions and local languages. The binomial nomenclatureMode of scientific designation of animal and plant species consisting in following the genus name by the qualifier of the species name. proposed by the Swedish botanist, physician, and zoologist Carl von Linné [3] made it possible to precisely name a given species. When established in the 18th century, species were considered as fixed entities that were defined by morphological criteria. Thus, Carl von Linné classified plants according to flower structure and more precisely the number, arrangement and proportion of the reproductive organs: stamen and pistil. However, the 19th century marked the end of this idea of fixed and eternal species. First of all, George Cuvier [4] (see Focus Georges Cuvier), realized that some animals existed but no longer exist: the great diversity of fossils originally unexplained were then described as extinct species, and this ranges from shells to dinosaurs (see FocusThe species for the palaeontologist).
All this work has led to the classic classification (or taxonomic rank) of living organisms based on observable characteristics and a hierarchy of categories defined as follows: (living) → Domain → Kingdom → Phylum or Division → Class → Order → Family → Genus → Species.
This hierarchy has been totally challenged by the notion of species evolution due to natural selection developed by Darwin (see Theory of Evolution:.. & Focus on Darwin), i.e. the filiation of species and their common descent from a universal ancestor. The term “natural selection” was coined by Darwin by analogy with artificial selection by farmers or herders who choose in each generation the individuals with the “best” characteristics to reproduce them. This revolutionary notion will make it possible to reflect an evidence recognized by all: within the same species, some are more similar than others, but all are different. I look like my parents, brothers or sisters, but I am different from them.
Since the second half of the 20th century, phylogenetic classification (see below) has developed in this way. It aims to account for the degrees of relationship between species and thus to understand their evolutionary history, or phylogenyStudy of the evolutionary relationships among individuals or groups of organisms (e.g. species, or populations)..
3. Intraspecific diversity, genetic diversity and species evolution
According to Darwin, each new generation of a given species is made up of individuals which, despite their similarity, have different abilities to survive in their environment. Each individual thus presents a unique combination of characteristics (physical, genetic, ability to adapt to the environment…) of the species to which he belongs. Facing the constraints and changes in their environment (climate, predation, parasites, resources, etc.), some will have difficulty to survive and reproduce and will eventually disappear. Others will adapt more easily and survive. They will then pass on their advantageous characters to their descendants.
All individuals of the same species, genetically related but different, living in a relatively small geographical area represent a population. When a population of a given species is geographically isolated, individuals will develop more or less rapidly if the living conditions are satisfactory. Generation after generation, they will develop characters or skills different from those of the original populations. We are talking about diversity within the same species or intraspecific diversity. The ultimate stage of this divergence is when individuals in the population become unable to reproduce with individuals of the original species. A new species is born (Figure 4). A classic example is that of Darwin finches (see focus Darwin’s finches always at the forefront). The importance of geography in the speciationEvolutionary process at the origin of the appearance of new living species individualized from populations belonging to an original species., already imagined by Alfred R. Wallace [7] in the 19th century, will be used by Alfred Wegener [8] when he puts forward, in 1915, the hypothesis of continental drift (ancestor of the current plate tectonics [9]). Having found that several animal and plant fossil species were very similar on the American and African continents until the beginning of the secondary era (-200 million years ago), from which time fossils diverge on each of the continents, Wegener then imagined that the latter were formed from the bursting of a super-continent, the PangaeaSupercontinent formed in the Carboniferous period from the collision of existing continents on the Earth’s surface and which then regrouped all the emerged lands. In the Triassic, it split into two continents: Laurasia in the north and Gondwana in the south.. A classic example is that of birds from the Ratites group: African ostrich, South American rheas, Australian emus, New Zealand Kiwis that differentiated themselves from an ancestor, a kind of “paleo ostrich”, spread all over the Pangaea.
As Darwin developed his theory, Mendel [11] discovered how the characters of a living organism were transmitted from generation to generation. This discovery, which went unnoticed at the time, was at the origin of the development of genetics, which made it possible to understand the mechanisms at the origin of the evolution of species. The integration of genetics into Darwin’s theory gradually took place in the first half of the 20th century. However, it was not until 1953, when Watson and Crick discovered the structure of DNA (deoxyribonucleic acid) [12] and characterized its functioning that DNA recognized its role as a carrier of heredity and marker of the progeny of a living organism. The properties of the genome of living organisms (see The genome between stability and variability) will then be dissected, as will be the mechanisms leading to mutations and recombinations affecting individuals and essential for the adaptation of populations to environmental changes, i.e. for evolution. Intraspecific diversity is in fact genetic diversity. Variations induced by genetic mutations are responsible for polymorphismLinked to variations induced by genetic mutations, polymorphism refers to variations in the nucleotide sequence of a gene’s DNA in a population. It refers to the coexistence of several alleles for a given gene or locus in an animal, plant, fungal or bacterial population. of individuals (see Genetic polymorphism and selection). These mutations can be “neutral”, “slightly deleterious” or “favourable” from the point of view of natural selection. They are, or are not, preserved in the genetic heritage of the species or sub-population by different adaptations. Each species therefore has a unique combination of genes, but each individual of the same species will have characteristics that distinguish it from other individuals of that species.
An even more discrete level of regulation makes it possible to understand, for example, the differences existing in identical twins: it is epigenetic regulation (see Epigenetics, the genome and its environment) that concerns all modifications, materialized by biochemical modifications (a methyl group CH3, for example) of DNA and which are not encoded by the DNA sequence. They allow a different reading of the same genetic code and are expressed during the development of the organism. While some epigenetic marks are transient, others have remarkable durability and may even pass to offspring, but generally in a transient manner. However, since they are not based on changes in the DNA sequence, they do not modify the genetic structure of the line concerned and a speciation process is therefore excluded (see Adaptation: responding to environmental challenges).
4. The Tree of Life
5. Ecosystems
Classically, an ecosystem is defined as the whole formed by an association of living organisms (or biocenosis) and their biotope, i.e. the biological, geological, edaphic (soil), hydrological, climatic environment, etc. There are therefore an infinite number of ecosystems: a peat bog (see Peatlands and marshes, remarkable wetlands), a forest, a “black smoker” on the ocean floor (see Black smokers’ ecosystems) are well known ecosystems; but the rumen of a ruminant, a camembert or a decomposing organism also constitute different ecosystems (see the series of articles under the title “Ecosystems“). Broadly speaking, an ecosystem is therefore characterized by interactions (between living species and with the surrounding environment), flows of matter and energy between each of the components of the ecosystem allowing their life and a dynamic balance over time, between sustainability and evolution. In an ecosystem, the term ‘matter’ refers to all of the living (plants, animals, organisms) and nonliving (air, nutrients, water) things in that environment.
5.1. Species interactions in ecosystems
The networks of interactions and interdependencies that exist between organisms in the same ecosystem are the very essence of the concept of biodiversity (see Symbiosis and parasitism). These interactions are often mutually beneficial and their role in the physiology and adaptation of organisms is essential. For example, many animals cannot digest without the Bacteria and Archaea present in their digestive tract, most plants can only use the soil with fungi colonizing their roots, which they feed in return. This fungus/root association is called mycorrhizal symbiosis.
But this is not always the case: interactions between two organisms can be classified according to their beneficial, harmful or neutral nature for both partners. Thus, interactions that are beneficial for one partner and harmful for the other (predation, parasitism), beneficial for one and neutral for the other (commensalism) and mutually beneficial interactions (mutualism) can be distinguished, even if in reality all intermediate situations can exist, in a true continuum of interaction types. They can also be classified according to their instantaneous (predation) or sustainable nature (parasitism, mutualism, etc.), as well as according to the degree of association between the partners. Etymologically, the term symbiosis refers to “living together“. This definition refers to a sustainable and mandatory coexistence, involving all or part of the life cycle of the two organisms, regardless of the exchanges between them. A more restrictive definition reserves the term symbiosis for sustainable and mutualist coexistence (see Symbiosis and parasitism).
Thus, in an ecosystem, thousands of species coexist and extremely complex interactions are at the root of its general functioning, which is characterized by a functional biodiversity dynamics reflecting the consequences of all these interactions, such as the production of ecosystem servicesBenefits we obtain from ecosystems without having to act to obtain them. The various types of services are the result of natural processes of ecosystem functioning and maintenance. Thus, supply services provide the goods themselves such as food, water, wood and fibre. Regulatory services regulate climate and precipitation, water (e. g. floods), waste, and the spread of disease. Cultural services are about beauty, inspiration and recreation that contribute to our well-being. Assistance services include soil formation, photosynthesis and recycling of fertilizing substances, without which there would be no growth or production. (see Biodiversity is not a luxury but a necessity).
5.2. Flow of matter and energy through ecosystems
The matter follows the law of the conservation of mass enunciated by Lavoisier “Nothing is lost, nothing is created, everything is transformed“. Thus, in the example presented in Figure 7, the limnea, a kind of herbivorous aquatic snail, grazes a wide variety of aquatic plants and algae, all primary producerslive beings capable of producing organic matter from mineral matter, for example through photosynthesis. They are autotrophic organisms, located at the base of the food chain. They are ingested by a primary consumer, itself being the possible target of secondary consumers, living in the pond. When feeding itself, the limnea recover the material contained in these foods. The limnea is therefore a primary consumerA living being who needs to consume other living beings to produce his own organic matter in order to grow and grow. It is a heterotrophic organism. Herbivores, which only consume terrestrial or aquatic plants, are primary consumers., that feeds almost exclusively on autotrophic organisms. The carp that feeds on many debris and small animals, and in particular on limnea, is a secondary consumerHeterotrophic living being that consumes organisms that are themselves consumers. This is typically the case for carnivorous predators (wolves, lions, etc.), which only feed on other animals and are at the top of the food chain.. This is also the case of a formidable carnivore, the pike, which feeds on various prey: various species of fish, amphibians, lizards, ducklings, rodents…, in turn recovering the material that constitutes them. Around the pond, especially on the banks, birds of prey – like the marsh harrier – can easily find their food: small mammals, frogs, fish, insects and birds, for example.
In the same way, energy also flows through an ecosystem. In meadows, forests or ponds, sunlight is the primary source of energy. It is the autotrophic organisms [21] that transform the Sun’s light energy into chemical energy through photosynthesis. This energy is used by the plant to produce organic matter from water, environmental minerals and CO2 from the atmosphere. By eating this organic matter, consumers, heterotrophic organisms such as limneas, frogs, pike or harriers, can then recover the energy produced by plants. A large part of this energy will be lost: some in waste, some in heat, but the rest of the energy will be used and will allow it to grow, develop and reproduce. Energy transfer thus continues throughout the food web.
There is always a loss of energy from one trophic level to another. Since the energy of an ecosystem is not recycled, its functioning requires that it be continuously supplied with energy from an external source, such as light for photosynthesis. In other ecosystems such as hydrothermal sources on the ocean floor, autotrophy is provided by a process where energy is not provided by light, but by chemical molecules: this is chemosynthesis (see Microbes in extreme environments).
5.3. Ecosystem dynamics
Ecosystems are dynamic entities controlled by both external and internal factors. External factors, such as climate and soil type, control the overall structure and functioning of ecosystems. Thus, each ecosystem has specific environmental conditions (temperature, humidity, pH, soil minerals, etc.) that allow plant, animal or microbial populations to live, interact and develop through the transfer of matter and energy. Conversely, the species present shape the ecosystem, which thus evolves over time. These relationships ensure that each population of individuals present has the conditions and resources necessary for their survival. Soil is therefore essential within a terrestrial ecosystem. Not only is it the substrate on which primary producers are fixed, but it provides a diversity of habitats (e. g. burrows) for many animals, and it also acts as an accumulator, processor and transfer medium for water and other products supplied, in particular minerals.
While resource inputs are generally controlled by external processes, the availability of these resources within the ecosystem is controlled by internal factors such as the decomposition of organic matter, the distribution of living species, competition between root systems, etc. From one year to the next, biotic environmentsrelated to life. The biotic factors of an ecosystem are the flora and fauna and the relationships between them. The environment in which life can develop. and abioticsPhysical and chemical factors in an ecosystem that influence a given biocenosis. Opposable to biotic factors, they constitute part of the ecological factors of this ecosystem. Climatic factors (temperature, light, air…), chemical factors (air gases, mineral elements…) are abiotic factors. ecosystems can vary. A severe drought, a particularly cold (or mild) winter, or a pest outbreak mean that animal populations will vary greatly from year to year. They increase during periods of abundance, but collapse when the food supply becomes difficult. Thus, pollution, drought, temperature changes, but also disease development, can affect the ecosystem, and it is the diversity within the various populations inhabiting the ecosystem that will allow, or not, organisms to survive these disturbances.
A spectacular example is the succession of plants in a forest that is opened by thinning or destroyed by severe storms or fires (Figure 9). The cycle starts from a so-called pioneering stage and tends to reach an equilibrium stage, the climax, until a disturbance (fire, windstorm, flood, landslide, avalanche…) reintroduces the conditions of the first stage. In the example described in Figure 9, the forest is brutally destroyed by fire to ground level. Seeds, present in the soil or brought by wind, water or animals, will germinate. The first grasses and other herbaceous plants that grow back are called pioneers, so small bushes and young trees begin to recolonize the area. In this new stage, conifers grow quickly and hardwoods grow more slowly in their shade. Large evergreen or deciduous trees “densify” the canopy while shade intolerant species disappear as the forest grows. At the end of the cycle, the ecosystem returns to a state similar to the one in which it began, until the next disturbance. Thus, as this succession of more or less catastrophic events unfolds, plant communities and the associated microbial, fungal and animal communities evolve by replacing each other.
Indeed, ecosystems have varied considerably over millions of years of evolution and adaptation: a peat bog in the Carboniferous period probably has nothing to do with a mid-mountain peat bog in the Jura; but the principles that governed their respective functioning are most probably identical (see The first complex ecosystems & Peatlands and marshes, remarkable wetlands).
Nowadays, an additional element must be taken into account with the intervention of a single living species, Homo sapiens (see Biodiversity is not a luxury but a necessity). Indeed, the threats we pose to biodiversity are particularly numerous. A first category of threats concerns the fragmentation, destruction or modification of ecosystems through increased agricultural activities, overfishing and various forms of pollution. The artificialization of territories, with various constructions, roads, car parks, buildings, etc., also weighs heavily on ecosystems. Finally, this is also the case for the development of transport and trade, which in particular promotes the development of invasive species, such as the Asian hornet (see Climate change and globalization, drivers of insect invasions). Ecosystems can then completely lose their resilience. More generally, human activities modify, or even significantly disrupt, our environment, ranging from landscapes to natural biogeochemical cycles (see Carbon cycle disrupted by human activites & Nitrates in the environment).
Thanks to Catherine Lenne, Professor at the UMR PIAF (INRA and Blaise Pascal University) in Clermont-Ferrand who knows everything about the daily life of plants (and much more), for her critical eye and advice when writing this text.
References and notes
Cover image. Africand Jacana (Actophilornis africanus) walking on water lily leaves, Baringo Lake, Kenya [source: © Jacques Joyard]
[1] Lecointre G (2011) Les espèces, c’est nous qui les faisons ! Espèces 1, 68-72 (in french)
[3] Carl von Linné was know as Carl Linnaeus, 1707-1778, before his ennoblement; Biography on Wikipedia
[4] Georges Cuvier, 1769-1832; see focus Georges Cuvier
[5] http://www.florenum.fr/ (in french)
[6] http://www.desfleursanotreporte.com/ (in french)
[7] Alfred R. Wallace, 1823-1913; http://people.wku.edu/charles.smith/wallace/BIOG.htm
[8] Alfred Wegener (1880 – 1930); Wikipedia
[9] http://planet-terre.ens-lyon.fr/article/derive-continents-wegener.xml (in french)
[10] Lecointre G. (2009) Guide critique de l’évolution », Belin (in french)
[11] Johann Gregor Mendel (1822-1884); Wikipedia
[12] James Watson (born 1926) & Francis Crick (1916-2004); Biographies (as well as those of Rosalind Franklin, 1920-1958, & Maurice Wilkins, 1916-2004) on the website: http://www.medecine.unige.ch/enseignement/dnaftb/19/concept/index.html
[13] Guyader H & Lecointre G (2013) La classification phylogenetique du vivant. Belin Paris, 608 p., ISBN 2701134560; http://www7.inra.fr/dpenv/leguyc46.htm
[14] Note that this ancestor remains hypothetical; he can only be identified by a few characters that he possesses and transmits to his descendants (the shared characters observed on the descendants), as puzzle pieces, but we do not have the complete “picture”.
[15] http://planet-vie.ens.fr/content/classification-vivant-mode-emploi (in french)
[16] It should be noted that the characteristics taken into account are so numerous and so heterogeneous that it is always necessary to choose, and depending on which ones are retained or eliminated, we end up with different cladograms, or trees. In this case, preference is given to the tree with the fewest knots (i.e. the most parsimonious).
[17] Woese CR & Fox GE (1977) Phylogenetic structure of the prokaryoticdomain: The primarykingdoms, Proc. Natl. Acad. Sci. USA 74, 5088-5090.
[18] Hug LA et al (2016) A new view of the tree of life, Nature Microbiology 1, #16048; doi:10.1038/nmicrobiol.2016.48
[19] http://www.fondation-lamap.org/fr/page/10998/la-classification-des-tres-vivants-principes-g-n-raux (in french)
[20] http://www.academie-en-ligne.fr/Ressources/7/SN12/AL7SN12TEPA0013-Sequence-07.pdf (in french)
[21] Autotrophy: The ability of an organism to produce organic matter from the reduction of inorganic matter and an external energy source: light (photoautotrophy, as in the case of photosynthesis) or chemical compounds (chemoautotrophy).
The Encyclopedia of the Environment by the Association des Encyclopédies de l'Environnement et de l'Énergie (www.a3e.fr), contractually linked to the University of Grenoble Alpes and Grenoble INP, and sponsored by the French Academy of Sciences.
To cite this article: JOYARD Jacques (March 27, 2022), What is biodiversity?, Encyclopedia of the Environment, Accessed July 27, 2024 [online ISSN 2555-0950] url : https://www.encyclopedie-environnement.org/en/life/what-is-biodiversity/.
The articles in the Encyclopedia of the Environment are made available under the terms of the Creative Commons BY-NC-SA license, which authorizes reproduction subject to: citing the source, not making commercial use of them, sharing identical initial conditions, reproducing at each reuse or distribution the mention of this Creative Commons BY-NC-SA license.