Air pollution particles: what are they?
PDF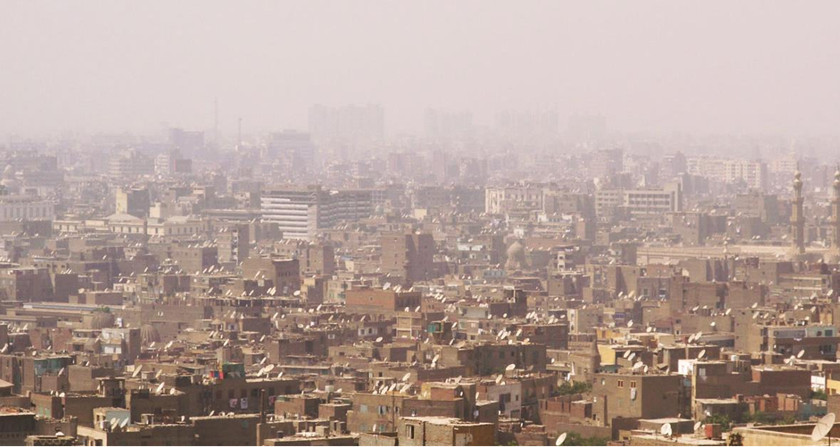
Particulate matter is a major component of air pollutants. They are considered to be one of the main causes of pollution-related mortality. Their finely divided state covers designations as well as physical and mechanical properties that are often poorly understood. This article aims to provide a better understanding of what particulate matter in the atmosphere means and the problems it raises, particularly in terms of quantifying its effects on health.
1. What are we talking about?
1.1. Particles and aerosols
1.2 Terminologies used to differentiate between particles
Different terms are used to describe particular types of aerosols, often incorrectly.
- Dust is a solid particle, usually greater than 1 µm, suspended by mechanical processes such as wind erosion (examples: sandstorm, ash, road dust, pollen).
- The term smoke refers to generally finer particles resulting from combustion. In English, fume also refers to very fine particles resulting from the condensation of a vapour.
- Fogs (fog and smog) are formed by a suspension of liquid droplets resulting from the condensation of a vapour, or from a spray. In the atmosphere near the ground, the term fog is used when visibility is reduced to less than 1 kilometre.
- When visibility is reduced to less than 1 kilometer,particles often have a size greater than 5 µm.
- Mists (mist or haze), are made up of smaller droplets or solid particles. They correspond to a lower visibility disorder than fogs, except in exceptional cases of haze in desert or semi-desert regions.
It should be noted that the English language is richer in this field than French. The French word “brume” can be translated into haze or mist. Smog is the contraction of smoke and fog. The translation in French is “brouillard photochimique”.
1.3. The acronyms PM
PM 10, PM 2.5 (PM is an abbreviation of Particulate Matter) are particles present in the atmosphere whose size is respectively less than 10 and 2.5 µm. These definitions have been introduced to take into account the behaviour of particles during inhalation. Indeed, particles with a size greater than 10 µm are mostly stopped in the nose and are not considered respirable. PM 2.5 is still called fine particles and those between 2.5 and 10 µm coarse particles. We will see later that these dimensions are in fact “cut-off diameters” (paragraph 4.2). About 50% of the particles are smaller than the cut-off diameter.
1.4. Other terminologies
Cloud Condensation Nuclei (CCN) nuclei are the germs around which water vapour condenses in the atmosphere. They have dimensions greater than a few tenths of a micron.
Black fumes are atmospheric particles measured by collecting them on a filter and determining the reflectivity of the filter by optical method. This measurement method has long been used to measure air pollution. Soot is carbon particles, usually from combustion, on which hydrocarbons may have condensed. Particulates from diesel engines have a high proportion of soot.
Particles smaller than 0.1 µm are called Aitken nuclei (an expression little used today), nanoparticles, or ultrafine particles (read Wood Combustion and combustion engines in accusation).
2. Particles in the atmosphere
2.1. Primary and secondary pollutants
Particulate matter includes both primary and secondary pollutants (read Air pollution). They can be emitted naturally into the atmosphere, but they can also be formed as a result of chemical or photochemical reactions between gaseous pollutants or by condensation of vapours in the atmosphere. Primary sources are very diverse, their origin being due to human activities but also natural with particles from volcanoes, wind erosion, sea spray, pollen, plant debris, etc. Through his activities, man can modify these natural emissions.
2.2. Some effects on the atmosphere
Particles modify the optical properties of the atmosphere by scattering or absorbing light. One of the manifestations of pollution is thus the reduction of visibility. Particulate pollution also affects the radiative balance of the atmosphere by backscattering or absorbing solar and infrared energy from the ground or the atmosphere. Particles are involved in the formation of clouds and fogs. Without them, condensation and freezing, resulting in water drops or ice crystals, would occur at lower temperatures. Homogeneous condensation, i.e. without a condensation nucleus, requires an oversaturation of 500%, homogeneous sublimation a oversaturation of 3000%, homogeneous freezing a temperature of -40°C. On the contrary, in the presence of particles, condensation can occur at a humidity below 100%. The number of droplets in a cloud and their size are thus influenced by the presence of particles that act as condensation nuclei. Continental clouds develop over a spectrum of nuclei that are more numerous and smaller than those of maritime clouds. They have more and smaller drops that make them less able to precipitate than maritime clouds made up of fewer and larger drops.
2.3. How are they characterized?
To know the effects of particulate pollution, depending on the objective pursued, it will be necessary to characterize more or less precisely:
- The concentration which, depending on the case, will be expressed in mass or surface area or in number per unit volume.
- The chemical nature of particulate matter, but in air pollution monitoring networks it is only the mass concentration that is systematically measured. It is the latter that is used in epidemiological studies.
- The properties of particles depend on their size. The distribution according to size is called the size spectrum (see section 3). Measuring the entire spectrum requires expensive and sophisticated equipment.
2.4. What is particle size?
When we talk about the size of a spherical particle, it is either its radius or more frequently its diameter.
Several definitions of particle size apply when particles can be observed by optical or electron microscopy and have any shape. The most common dimension is the diameter of the circle in which the particle can be included or at least its projection in the observation plane.
Very often particles cannot be observed under a microscope for various reasons. First of all, at the time of collection on a medium, there is a risk of a change in the particle size. The presence of condensed vapours on their surface or in volume also modifies the balance between the particle and the air in which it is suspended. Electron microscopy requires that the particles are placed in a vacuum and then bombarded by electrons, which very quickly produces the evaporation of condensed vapours and thus modifies the characteristics of the particle. Finally, the use of optical microscopy is limited to objects a few tenths of a micrometre in size.
To determine the particle size, we use the measurement of a physical characteristic of the particle that depends on this dimension: its thermal agitation coefficient (or Brownian motion), light scattering, mobility in an electric field, sedimentation rate, etc. The spherical particle is assumed and the diameter equivalent to this physical characteristic is obtained.
Particulate matter is often characterized by an average size, but different definitions of the average can be used without being clearly specified by the authors. This may be the arithmetic mean or the geometric mean, the definitions of which are standard in statistics.
3. Granulometric spectra
The particle size spectrum is represented by a distribution function that expresses the fraction of mass (dM) or surface (dS) or number (dN) in a small range of dimensions dD as a function of dimension D (e.g. diameter). The distribution functions are generally normalized, i.e. the values dM, dS, dN are divided by the total mass M or the total area S or the total number N.
The mass, surface or number distributions will be represented by very different curves (Figure r). Since particles are distributed over several orders of magnitude, for example between one hundredth of a micrometre and a few tens of micrometres, which is the case with aerosols, none of the three distributions can by itself adequately represent the entire distribution.
Depending on the part of the grain size spectrum that you want to identify, it is therefore necessary to measure the number, or mass, or surface of the particles. Surface determination is relevant when considering the chemical reactivity of particles in a gas, since this reactivity depends on the surface of the particles. The choice of particle size distribution and consequently the method of particle measurement is essential.
If it is accepted that the finest particles present a greater risk to health, the measurement of mass concentration alone is not an appropriate characteristic; then number and mass measurements should be combined. Now, Euro 6 standards (which regulate engine emissions) take into account the number of nanoparticles (read the articles Diesel engines and wood combustion in charge, Air pollution: Understanding to inform and prevent and How does the law protect air quality). However, these emissions are not measured in air quality monitoring networks.
4. Main properties of particles
The properties of a particle are much more complex than those of a gas molecule. In order to understand the behaviour of particles and their toxicity, it is essential to know some of their main mechanical and physical characteristics: falling velocity under the effect of gravity, inertia, thermal agitation or Brownian diffusion. These characteristics play a very important role in the deposition of particles in pipes (e.g. in the respiratory system), in their filtration or in the coagulation of particles between them.
4.1. The sedimentation rate
The rate of fall of particles suspended in a gas (not turbulent) under the action of gravity is called the sedimentation rate. The particle subjected to its weight and Archimedes’ thrust quickly reaches a vertical velocity that is called the sedimentation velocity. This speed depends on the square radius and the specific weight (or density ρ) . In general, we do not know the density of the particles or their shape. We assume the particles spherical with ra is the aerodynamic radius. It is the radius of an equivalent particle, with a density equal to 1, which has the same sedimentation rate as the actual particle whose density is not known. The sedimentation rate only becomes appreciable for particles with a radius greater than one micrometer. It is important (about 1000 m/h) for particles with a radius of 50 µm (diameter 100 µm). Beyond this dimension, particles can no longer be considered as suspended in the gas and we no longer talk about aerosols.
4.2. Inertia
4.3. The Brownian diffusion
It is the irregular movement of particles resulting from their collisions with the molecules of the gas. Table 1 shows the average displacement in one second, projected on an axis. This displacement becomes appreciable and more important than that due to gravity when the aerodynamic radius of the particle becomes less than 0.1 µm. This is an important property of small particles. In an enclosure, in a pipe, in the respiratory tract, because of this Brownian agitation, the particles will settle on the walls. In a filter, very small particles are effectively stopped because of this agitation and they are more easily collected when they are small, which may seem paradoxical. It is often stated, but wrongly, that very small particles are difficult to stop.
Table 1. Comparison between Brownian agitation and sedimentation
Table 1 shows a comparison of the velocities (in cm/minute) of spherical particles with a density of 1 : on the one hand due to Brownian agitation (second line of the table) and on the other hand due to the sedimentation rate (third line) according to Fuch [3], under normal temperature and pressure conditions. When they are very small, the displacement due to Brownian agitation becomes greater than that due to the falling velocity. Diffusion then becomes more important than sedimentation.
In a turbulent gas, such as the atmosphere near the ground, the turbulent agitation is several orders of magnitude greater than Brownian agitation. It does not depend on particles but on the medium and the flow. Subjected to this turbulence, particles can travel thousands of kilometres, despite their sedimentation rate. Thus the Sahara wind spray is found in Europe or on the other side of the Atlantic Ocean (see Figure 1).
4.4. Coagulation
It is the process of adhesion or fusion of particles between them. The smaller the particle size, the stronger the adhesion. It is indeed intermolecular forces with a very short range of action, such as Van der Waals’ force, that are involved. Coagulation results essentially from Brownian motion, which causes particles to collide. It thus leads to a reduction in the number of particles in the aerosol and an evolution of the particle size spectrum, in the direction of particle magnification. The density remains constant. Coagulation is an important phenomenon when the number of particles is high. It is all the more effective when the particles have different dimensions. Thus, with identical particles, for which the phenomenon is the least intense, it takes 33 minutes to halve the numerical concentration of an aerosol with 106 (one million) particles per cm3 and 5.5 hours when the initial concentration is 105 particles per cm3.
As a result of this coagulation phenomenon, the finer particles cannot persist in an aerosol in the absence of continuous production, and their lifetime is shorter the higher the numerical concentration.
5. Some consequences of mechanical properties
5.1. The sampling
The sampling of aerosol particles should therefore be carried out with care in order to limit the uncertainties inherent in the mechanical properties. It should be added that gases such as water vapour or organic products can condense on the particles but also evaporate when the particle is collected. The collected particles should be as close as possible to the aerosol analyzed (Figure 5).
5.2. Deposition in the respiratory system
Figure 6 shows the retention of particles according to their size and the different parts of the respiratory system:
- The largest particles are stopped by inertia in the nasopharyngeal segment, but the smallest (less than 10-2 µm) are also stopped by Brownian diffusion.
- Particles smaller than 0.5 µm penetrate the bronchi and lungs where some of them are deposited.
- Above 10 µm the particles stopped by the nose do not enter the respiratory tract, bronchial tubes and lungs.
- Breathable particles have a diameter of less than 10 µm [4], [5]. The finest particles are stopped by Brownian diffusion, especially in alveolus.
- Between 0.1 µm and 1 µm the inertia phenomena are no longer as important as for the largest particles and diffusion is not yet effective.These fine particles can therefore penetrate deep into the respiratory tract, but they are also the least retained. This highlights the link between the mechanical properties of particles and the risk of their deposition in the respiratory tract [6].
6. Optical properties
The optical properties of particles make it possible to understand the visibility problems that accompany pollution, giving some qualitative information about the aerosol. They are used to measure the dimensions and concentrations of particles, particularly from satellite observations [7].
Aerosol particles diffuse light. When the particle size is small in relation to the wavelength of the radiation, i.e. particles with a diameter of less than 0.1 µm, the scattering is called Rayleigh scattering (se The colours of the sky). The total radiated energy is proportional to R6/λ4 where R is the radius of the assumed spherical particle and λ is the length of the radiation. Short-wavelength radiation is much more strongly scattered than long-wavelength radiation. For the same flow, blue is about eight times more widespread than red. The blue of the sky, of a calm sea, are a consequence of the Rayleigh diffusion on the molecules of air or water; likewise the bluish grey aspect of cigarette smoke or certain mists made up of very fine particles.
Cigarette smoke illustrates the effect of particle size on the appearance of smoke. Indeed, when it expires it becomes whiter in appearance, due to the increase of particles size with water vapour in the respiratory tract. This change in optical properties clearly shows the change in size of some particles with the humidity of the air. This point is important for measuring the mass concentration of particles (read Diesel engines and wood combustion in charge). Observation of the atmospheric aerosol gives an idea of the origin of the particles. If they come from a gas-solid transformation, the aerosol has a blue haze appearance. If they come from wind erosion (case of particles coming in Europe from Sahara) the aerosol is whitish in colour (see paragraph 7.1).
At sunrise or sunset, the light coming from the sun is reddish in appearance (Figure 7). This is due to the lack of short wavelengths (blue for example) that have been scattered and are then absent, leaving the emphasis on red light. When the particle size becomes comparable to the wavelength, or larger, the scattering is preferentially in the direction of the incident light. It is less sensitive to the wavelength of radiation. A cloudy sky or one with particles larger than one micrometre becomes white or whitish. The amount of scattered light does not vary as much with the size of the particle.
Aerosol particles are also made up of substances such as carbon, which can absorb light. This phenomenon becomes prevalent with infrared radiation emitted by the ground or the atmosphere[8].
7. Consequences of optical properties
7.1 Action on visibility
7.2. Action on the ground-atmosphere radiation balance
Aerosol particles play a complex role in the radiation balance of the atmosphere. Indeed, they diffuse and absorb part of the sun’s rays, the absorption occurring mainly in the infrared part. This leads to a backscattering towards space and a cooling of the planet. In addition, particles also absorb a fraction of the infrared radiation from terrestrial terrestrial sources, which results in a contribution to the greenhouse effect with warming of the lower atmosphere. The combined effects of these two mechanisms depend on the characteristics of the atmospheric aerosol but also on the properties of the soil and its reflection coefficient of solar radiation (called albedo). (read The Atmosphere and the Earth’s Gas Envelope). This is one of the reasons why it is difficult today to accurately quantify the effects of particulate matter on the planet’s radiation balance. It is generally accepted that particles of anthropogenic origin slow down the increase in temperature due to greenhouse gases.
8. Messages to remember
- Airborne particles are one of the components of the complex mixture called an aerosol.
- Their dimensions cover a very wide range of orders of magnitude, from a few nanometers (ultrafine particles) to a hundred microns.
- Their physical properties (sedimentation, inertia, coagulation) and their ability to settle, both in sampling systems and in the respiratory system, vary greatly with their size.
- The measurement of mass concentrations does not allow ultrafine particles to be taken into account.
- The abundance of particles in the air results in fairly strong optical properties, affecting in particular its transparency and reducing visibility.
- The radiation balance of particles is complex. By absorbing part of the earth’s infrared radiation, they contribute to the greenhouse effect and global warming. But, conversely, by backscattering part of the solar radiation back into space, they contribute to its cooling.
References and notes
Cover image. Smog in a polluted city (Cairo). [Source : By Sturm58 at English Wikipedia (Transferred from en.wikipedia to Commons.)[GFDL (http://www.gnu.org/copyleft/fdl.html) or CC BY-SA 3.0 (https://creativecommons.org/licenses/by-sa/3.0)], via Wikimedia Commons]
[1] The logarithm is the mathematical operation that substitutes an addition for a multiplication: log(ab)=log(a)+log(b). Multiplying a quantity by 10, a number for which the decimal logarithm is the unit, then results in the addition of a unit to its logarithm. In the usual notations, log10 refers to the decimal logarithm, while Log refers to the naperian logarithm, whose base is the irrational number e = 2.71828..
[2] According to Whitby K.T., Sverdrup G.M. Adv. About. Science Technology, 9, 477-525, 1980
[3] Fuch N. The mechanics of aerosol, Mac Millan, New York 1964
[4] Task group on lung dynamics. Health Phys. 12, 173, 1966.
[5] ICRP. Human respiratory tract model for radiological protection. Ann. ICRP 24, 1-3, 1994
[6] For more information on the relationships between particle properties and health effects, see the article Particulate Matter and Health.
[7] http://www.lmd.polytechnique.fr/~menut/documents/2016-LaMeteoSatAQ-REVetFIGS.pdf
[8] Renoux A. Boulaud D. Aerosols. Physics and metrology. Lavoisier TEC and Doc, 1998
The Encyclopedia of the Environment by the Association des Encyclopédies de l'Environnement et de l'Énergie (www.a3e.fr), contractually linked to the University of Grenoble Alpes and Grenoble INP, and sponsored by the French Academy of Sciences.
To cite this article: FONTAN Jacques (January 15, 2021), Air pollution particles: what are they?, Encyclopedia of the Environment, Accessed April 26, 2024 [online ISSN 2555-0950] url : https://www.encyclopedie-environnement.org/en/air-en/air-pollution-particles-what-are-they/.
The articles in the Encyclopedia of the Environment are made available under the terms of the Creative Commons BY-NC-SA license, which authorizes reproduction subject to: citing the source, not making commercial use of them, sharing identical initial conditions, reproducing at each reuse or distribution the mention of this Creative Commons BY-NC-SA license.