Atmospheric halos
PDF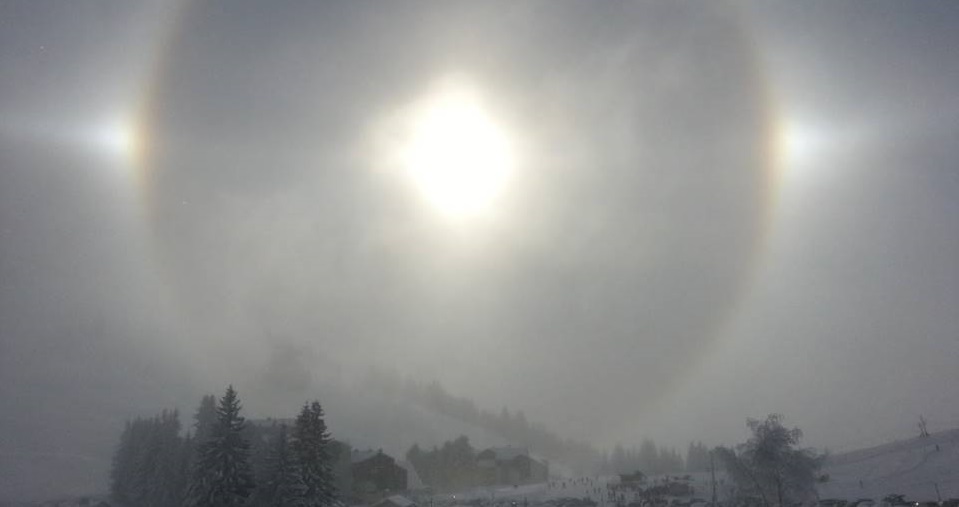
In the Earth’s atmosphere, light often offers a spectacle that can be appreciated simply by looking at the sky with the naked eye. In a generic way, atmospheric light phenomena are called photometeors, from the Greek words “photo” and “meteora” which mean respectively “light” and “which is in the air” [1]. The rainbow and the glory (read the focuses Spectacular Rainbows and Brocken’s Amazing Spectrum), which result from the interaction of light with water drops, are well known examples. Ice crystals also produce photometeors called atmospheric halos. Etymologically, the term “halo” refers to an aureole [2], viz., here, a luminous circle surrounding the Sun, the Moon or, possibly, any other light source. Broadly speaking, an atmospheric halo is a more or less strong accumulation of light, appearing in the sky as a spot, a circle, or an arc, which is mainly due to the refraction and/or reflection of light by ice crystals. There is a wide variety of halos, some of them are frequent, while others are much rarer and often only predicted. Sometimes coloured, their observation informs us about the properties of ice crystals in the atmosphere.
The first observations of halos date back to Antiquity, but it was not until the 17th century that a scientific approach (synthetic, explanatory and predictive) is developed with the work on Optics by Descartes [3] and Huygens [4]. A boost is then given in the 18th and 19th centuries with more and more precise observations and thanks to detailed studies by physicists such as Arago, Babinet, Bravais, Mariotte, Venturi and Young. It is worth mentioning that Bravais is the author of a complete thesis [5] on atmospheric halos known in the 19th century.
1. Some essential properties of ice
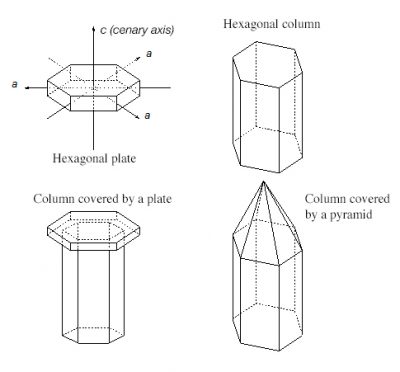
Despite the great variety of their shapes, four types of crystals are sufficient to explain the majority of atmospheric halos (Figure 1): the hexagonal column and plate, the column capped with a platelet and the so-called rifle bullet shape (a column capped by a pyramid). These crystals have a regular hexagonal geometry, with more or less smooth faces that constitute diopters [20] for light and have four axes of symmetry: three coplanar axes (a) passing through the vertices of the hexagon and forming two by two an angle of 120⁰, then the senary axis [6] (c), which is perpendicular to the hexagonal plane.
In the atmosphere, the smallest crystals, smaller than about 50 microns in size, have a Brownian (or random) motion and therefore do not have a preferential orientation. Between 50 and 500 micrometers, the gravity and the aerodynamic friction are the dominant forces and so the orientation that offers the least air resistance is generally favoured. Beyond these sizes, the large crystals whirl around a horizontal axis. These behaviours, combined with specular reflections [21] and refractions [22] by crystalline faces, explain the halos diversity. It should be noted that several types and sizes of crystals coexist in the air; different halos then often appear simultaneously in the sky and form what is called a halo system.
Furthermore, the ice has two refractive indexes, one along the a-axis and the other along the c-axis, which are very close from each other and slightly decrease from the purple to the red; their average value can be taken at about 1.31. This variation explains why halos formed by refraction are, in principle, iridescent. However, the red, the orange and the yellow are more intense than the green and the blue, at least for the obvious reason that the sky background is blue.
Although they are numerous and of different appearances, they can be classified in several ways: frequency of observation, shape, number of refractions and reflections (internal or external), degree of crystal orientation or, what amounts to the same thing, their size. It is this last possibility that is adopted here.
2. Halos produced by small crystals of random and equiprobable orientations
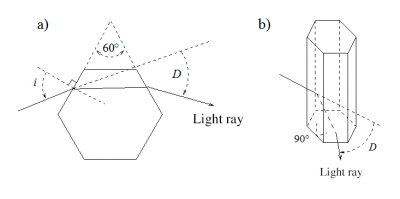
Halo at 22° – This is the most common (see the cover image of the article). It is a ring, centred on the Sun, with an angular radius very close to 22° and an angular width [23] of about 1 to 2°. Its inner edge is very clear, sometimes reddish, while its outer part is less frank and sometimes tinted blue pulling towards white (cover image). All these properties are explained by the deviation of light by the alternating lateral faces of a hexagonal crystal which constitute a prism of top angle 60° [7] (Figure 2a). This deviation reaches an absolute minimum around 22° where light accumulates (read Focus on Deviation of light by a prism). Due to the very large number of prisms presenting equiprobable orientations between 0 and 360°, the halo appears circular in the sky [8].
Halo of 46° – Similar to the previous one, this wider halo is however less frequent and more difficult to observe, on one hand because the light is redistributed over a larger portion of the sky and, on the other hand, because of unfavourable crystal height/width ratios. This halo involves orthogonal adjacent faces that form a right-angled prism (Figure 2b); the minimal deviation corresponding to such a prism is 46° (see Focus on Deviation of light by a prism). This explanation was initially suggested by Cavendish [9].
Unusual Halos – Other but rarer halos involve prisms from different angles. Theoretically, all values between 0 and 99.5° are possible, and so halos with an angular radius between 0 and 80° (read Focus on Deviation of light by a prism) can be observed. In practice, halos between 4° and 50° were observed: the halos of Van Buijsen (8°), Heiden (14°), Hall (17°), Dutheil (24°), Scheiner (28°), Feuillée (32°) and Burney (45°). These unusual halos are derived from prisms formed by crystals of more complex shapes: the pyramid (Figure 1) can explain some of these angles; for the Scheiner halo, it would be a very rare crystal with octahedral geometry [10]. Finally, let us mention the 90° halo, known as the Hevelius halo (1661), very little observed, which cannot be explained by means of prisms.
Note a trick to distinguish a halo from a corona which, like the halos above, is also a circular photometeor: as one moves away from the centre, the iridescence goes from red to purple for a halo; it is the opposite for a corona, because it is due to light diffraction.
3. Halos produced by intermediate size crystals
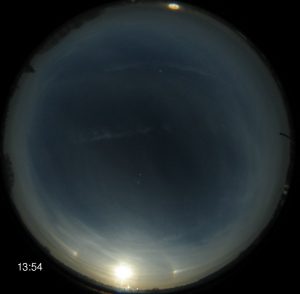
Parhelia – A parhelion [11], or false Sun, is a light spot located at the same height as the Sun and at a certain angular distance from it. They sometimes appear in pairs on either side of the Sun, symmetrically [12], [13].
The most common is close to the halo at 22°. We can see it accompanying the latter on the cover image, where two bright light spots are symmetrically arranged on each side of the Sun. Its formation involves the prism at 60° but whose edge is, this time, oriented vertically, like the senary axis. This orientation is decisive because the incident light rays are then oblique with respect to the main horizontal section of the crystal, which, after crossing it, concentrates the light at an azimuth distance greater than 22° (Bravais’ laws) [14]. This is why the parhelion spreads horizontally as it runs off the Sun (Figure 3); it is only when the Sun is low on the horizon that the parhelia are stuck to the halo at 22°. In addition, they are coloured, with red being closer to the halo than blue (Figure 4).
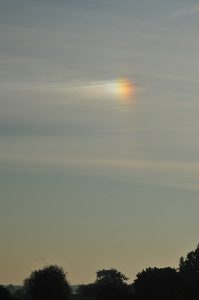
Some parhelia, much rarer, are located at an azimuthal distance from the Sun of more than 90° in absolute value. The best known examples are ± 120° °; the latter are not coloured. They are produced by an even number of internal reflections on the vertical surfaces of the crystals and by refraction on parallel surfaces (Figure 5). A parhelion between 150° and 160° was discovered in 1951 by the Swedish meteorologist Liljequist [15] in Antarctica. They are sometimes called paranthelia because they flank the point facing the Sun (azimuth angle 180°) called anthelion (“anti” means “opposite”). At this point, a very rare, uncolored halo may appear, whose process of formation is not certain.
The horizontal line on which the parhelia and the light source are arranged is called almicantarat; the latter is materialized, totally or partially, by a white light circle called a parhelic circle [16] if the light is reflected on vertical faces without privileged azimuthal orientation. Parhelia are sometimes spread vertically and then form so-called parelic arcs; they are attributed to possible small swings around the vertical orientation.
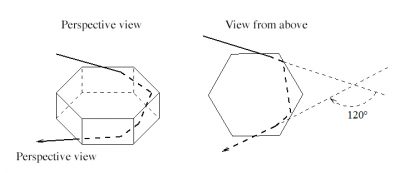
Light columns or pillars – These narrow vertical bands appear when the Sun is just above the horizon. They present the same colour as the source, and they are formed by reflections with a grazing incidence on the underside of the crystals of the senary axis slightly inclined with respect to the vertical. With perfectly oriented crystals, columns are thin and bright; any deviation from vertical alignment widens columns and reduces their brightness. From an airplane, one can see pillars under the Sun.
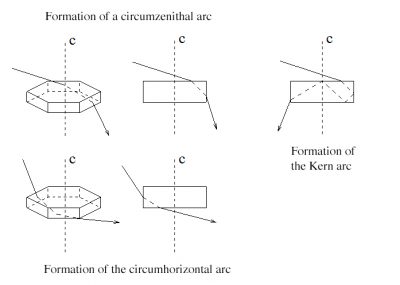
Circumzenithal and circumhorizontal arcs – These complementary arcs are bright and iridescent from the red to the blue as they progress towards the zenith or the horizon, respectively. They are formed by crystals with a vertical c-axis and a horizontal edge of the right-angle prism. They can accompany the halo at 46°. If light enters the crystal from the upper surface and emerges from a vertical surface, the arc is circumzenithal (or Bravais Arc, Figure 6); if it enters from a vertical surface and emerges from the lower surface, the arc is circumhorizontal (Figure 6). The first (Figure 7) partially surrounds the zenith and only exists if the Sun is at an angular height less than 32°. Beyond that, the reflection is total on the inner vertical side surface of the crystal. At 32°, the radius emerges grazing, so vertically, and the circumzenithal arc then appears as a bright point at the zenith. The arc is brightest if the Sun is 22° high; it touches the halo at 46° if it is present. The circumhorizontal arc, which partially surrounds the horizon and can therefore be masked by the landscape, behaves in a similar way: it only exists if the angular height of the Sun is greater than 58° and shines brightest for a solar angular height of 68° [17].
Parry’s arcs – These arcs are formed by crystals of horizontal c-axis with pairs of opposite faces, also horizontal. They are placed above and below the halo at 22⁰, with the concavity facing the halo; they go in pairs, but appear confused. They are named after the British Admiral Sir William Edward Parry [18] who explored the Arctic in the early 19th century and described many halos in his travel notes.
Sub-sun – From an aircraft, one can sometimes see an elliptical light spot under the horizon, symmetrical to the Sun; it is a sub-sun. It results from the reflection of sunlight by horizontal crystal faces. The sub-sun is circular if the crystals are perfectly aligned. This halo is occasionally haloed with a ring called Bottlinger’s ring.
4. Halos produced by large crystals
As they rotate, the large crystals form rare and complex halos whose appearance is highly dependent on the altitude of the Sun. These are arcs, lateral and tangential to the halo at 22° for some of them and 46° for others. They are iridescent in principle since they are formed by refraction.
Halo circumscribed to the 22° halo – It appears, when the Sun is close to the horizon, in the form of two arcs tangent to the 22° halo at its upper and lower points. These two arches lengthen as they approach each other when the Sun rises in the sky; they circumscribe the halo at 22° when the Sun reaches 30° high. As the Sun continues its ascent, this halo shrinks, becomes symmetrical, and eventually merges with the halo at 22° (Sun at 70° height); it is formed by the 60° prisms of columns with horizontal c-axis and rotating around it.
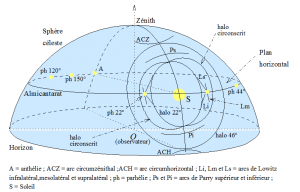
Lowitz lateral arcs- There are three and they pass through the parhelion at 22°: the supralateral arc and the infralateral arc are respectively above and below the Sun; the mesolateral arc is vertical through the parhelion. It was the German-Russian chemist Tobias Lowitz [19] who first observed them in St. Petersburg in 1790. These arcs are formed by the 60° angle prisms made up of plates rotating about an a-axis.
Tangent and lateral arcs of the halo at 46°- This time it is the 90° prisms that are involved. Tangent arcs have been predicted by numerical simulations; lateral arcs (supra and infra) have been poorly photographed and are rare.
5. Influence of some parameters
Up to now, one has considered crystals of regular geometry with assumed smooth enough faces, illuminated by a point source, and one has used the well-known laws of geometric Optics. If reality were that simple, we would see more halos. In fact, a cirrus is not always a homogeneous medium of crystals, as they often have rough surfaces that scatter light, irregular geometries, symmetry defects and internal impurities (aerosols, air bubbles) that disturb or even destroy the formation of halos.
The ice crystal density is also important: the light path can be modified by several crystals successively; it is the multiple scattering, which is responsible, for example, for parhelia at +/- 44° (a light beam deflected twice at ± 22° by two crystals). In addition, the light source (Sun, Moon) is wide; each of its points gives its own halo system. The latter overlap and the resulting halo appears wider, less clear and with faded colours. Finally, diffraction, important for small crystals, also tends to widen halos and tarnish their iridescence. The observation of a halo therefore reveals a lot of information about the degree of homogeneity of a cloud and the characteristics of its constituent crystals.
Halos is a vast subject of atmospheric optics, coupled with meteorology and ice crystallography. The variety of halos and their properties depend on the geometry of the crystals and their degree of orientation in space. Some of them remain uncertain or even of unknown explanation; this is the case, for example, of the elliptical halos and the Moilanen “V” arc which is halfway between the Sun and the upper part of the halo at 22°. What information about the crystals these halos bring us? Conversely, given an ice crystal geometry, which halos can be formed? Finally, what about other planets? Numerical simulation and observation are essential to answer these questions. Let’s hope that this article about some features of Earth halos will have aroused the reader’s curiosity about these beautiful photometeors and will encourage him/her to look up to the sky. May everyone be an attentive observer and sharpen their physical minds. Because we are all potential halo discoverers!
References and notes
Cover photo: Halo at 22° iridescent (clear inner edge, red, and more diffuse outer edge, bluish). Source: Hervé Herbin (LOA, Lille)].
[1] A. Rey: Historical dictionary of the French language. Ed. Le Robert (2012), 4200 p. (All the etymological notes in this article come from this dictionary.)
2] The word “halo” comes from the Greek “halôs” which refers to the area (disc) where wheat is threshed to separate the grain from the bale, hence by extension a circular surface and then a halo.
[3] R. Descartes: The dioptric. The meteors. In “Discours de la méthode, pour bien conduire sa raison et chercher la vérité dans les Sciences”, 1637, Ed. de Ch. Angot, Paris, 1668
[4] Complete works by Christiaan Huygens: Treatment of crowns and parhelia (De coronis and parheliis). 1662 or 1663. Dutch Society of Sciences, Volume 17
[5] Bravais A., 1847: Memoir on halos and the optical phenomena that accompany them. Journal de l’École Royale Polytechnique, Cahier 39, XVIII, Paris. This thesis, which has not really aged, offers a very detailed description, in terms of observation and theoretical explanation, and contains a large number of historical references.
[6] This axis is called senary because the crystal is found six times in the same configuration by a 360° rotation around this axis.
[7] The explanation by this prism is due to Mariotte: Mariotte’s Works, Traité des couleurs, 1686
[8] This is an illustration of the Curie principle according to which consequences (halo geometry) have at least the symmetries of causes (equiprobabilities of crystal orientations).
[9] According to T. Young, A course of lectures on natural philosophy and the mechanical arts. 1807. Vol. II, p. 308 (” Mr. Cavendish suggested, with great probability, that… “).
[10] Whalley E. 1981, Scheiner’s Halo: Evidence for Ice in the Atmosphere. Science, 211, 389-390
[11] The Greek words “para” and “helio” mean “near” and “Sun”.
[12] See also the historical text: Bravais A., 1845: Notice on the parilia located at the same altitude as the sun. Journal de l’École Royale Polytechnique, Cahier 30, XVIII, Paris
[13] If the light source is the Moon, we speak of paraselenes (Selene is the goddess of the full moon in Greek mythology).
[14] Bravais’ laws concern the refraction of a light beam whose incidence is oblique, i.e. forms a non-zero angle θ with respect to the plane of the main section of a crystal. There are two laws: (1) The incident radius and the emerging radius are also inclined (preservation of the obliquity θ) ; (2) The incident radius projected on the plane of the main section is deflected according to Snell-Descartes law as if it met a prism of refractive index [1 + (n² – 1) / cos² θ ]1/2
[15] Liljequist, G. H., Halo-Phenomena and Ice-Crystals, in Norwegian-British-Swedish Antarctic Expedition, 1949-52, Scientific Results, Volume 2, Part 2A, Oslo, 1956
[16] This name is due to Babinet (Babinet 1837: Mémoire d’optique météorologique. Academy of Sciences, 638-648).
[17] The 58° and 68° angles are complementary to the 32° and 22° angles: 90°- 32° = 58° and 90°- 22° = 68°.
[18] Parry W.E., Journal of a Voyage for the Discovery of a North-West Passage from the Atlantic to the Pacific; Performed in the years 1819-’20, in His Majesty’s Ships Hecla and Griper … with an Appendix Containing the Scientific and Other Observations… London (1821)
[19] Lowitz T., 1794, Description of a remarkable meteor observed in St. Petersburg on June 18, 1790. Nova Acta Academiae Scientiarum Imperialis Petropolitanae, 8, 384-388
[20] Separation surface between two media with different optical properties
[21] The reflection of the incident beam is done in one and only one direction, in accordance with the Descartes laws. If the beam is reflected in several directions, the reflection is said to be diffuse
[22] Deviation of light when it passes through a dioptre
[23] An angular distance (radius, width, size, etc.) is the angle between two points of a circle whose center (or vertex of the angle) is occupied by the observer. We also speak of apparent distance
The Encyclopedia of the Environment by the Association des Encyclopédies de l'Environnement et de l'Énergie (www.a3e.fr), contractually linked to the University of Grenoble Alpes and Grenoble INP, and sponsored by the French Academy of Sciences.
To cite this article: PUJOL Olivier (July 16, 2019), Atmospheric halos, Encyclopedia of the Environment, Accessed April 26, 2024 [online ISSN 2555-0950] url : https://www.encyclopedie-environnement.org/en/air-en/atmospheric-halos/.
The articles in the Encyclopedia of the Environment are made available under the terms of the Creative Commons BY-NC-SA license, which authorizes reproduction subject to: citing the source, not making commercial use of them, sharing identical initial conditions, reproducing at each reuse or distribution the mention of this Creative Commons BY-NC-SA license.