Human microbiotas: allies for our health
PDF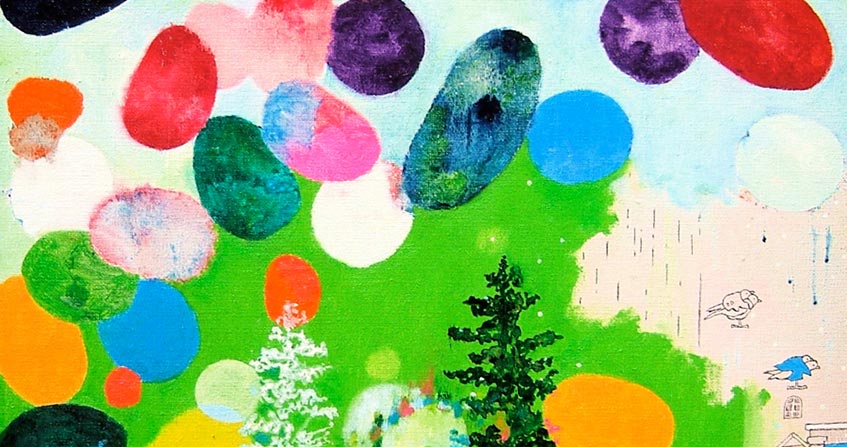
The human organism is home to a whole community of microorganisms: bacteria, archaea, yeasts and viruses. Today grouped under the term “microbiota”, they participate in many biological functions. We have thus developed a real mutualism with them. We host microorganisms in the skin (skin microbiota), in the mouth (oral microbiota), in the genitals (vaginal microbiota) and in the intestine (gut microbiota). We easily conceive of having bacteria in areas of contact with the outside world (skin, mouth). But the fact that our digestive tract also contains an impressive number of bacteria is much less intuitive, especially when it comes to very deep areas like the colon where there are more than1011 bacteria per gram of intestinal content. In total, our intestinal microbiota contains 100,000 billion microorganisms that contribute significantly to the proper functioning of our body and our health. Humans therefore represent a complex ecosystem composed of human cells and microorganisms. What if microbiotas are ultimately neglected organs?
- 1. Man and his microbiotas
- 2. Focus on the intestinal ecosystem
- 2.1. The birth of an ecosystem
- 2.2. Bacterial populations of the microbiota: a state of equilibrium
- 2.3. Human cells and bacteria participate in digestion
- 2.4. A microbiota/intestinal ecosystem: an essential barrier against intruders
- 2.5. Bacteria that educate our immune system
- 2.6. The microbiota/intestinal cells couple: a dialogue that can be heard throughout the body
- 3. Disruptions in the intestinal ecosystem: causes of the development of diseases
- 4. The microbiota, a therapeutic target of the future
1. Man and his microbiotas
Man does not host one but several microbiotas. Indeed, the same microorganisms are not found in all parts of the body and their proliferation depends entirely on where they are housed. Nutrient source, moisture conditions, presence or absence of oxygen, several factors influence the nature of the microorganisms capable of proliferating in a given area. Thus, we find in the body, a skin microbiota, an oropharyngeal microbiota, a vaginal microbiota or an intestinal microbiota. The niche occupied by each microbiota may be very small, such as specific skin areas, underarms or back skin.
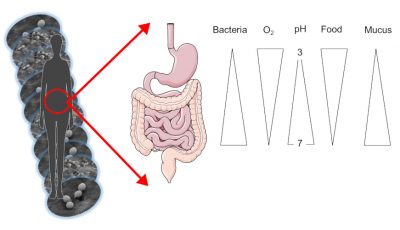
The skin microbiota feeds on sebumSubstance produced by small glands present in the skin. Sebum is made up of lipids. It acts as a protective layer on our skin. and dead keratinocytesCells mostly present on our skin.. It evolves both in wet areas, such as underarms or skin folds, and in drier but also oily areas such as the skin on the back. Similarly, our intestinal microbiota varies according to its location along the digestive tract (top or bottom). Indeed, the stomach is acidic and rich in oxygen while the colon, the terminal part of our intestine, is totally deprived of oxygen and acid-free.
The quantity of microorganisms, and in particular bacteria, also varies according to the area occupied: from about 1000 bacteria in the stomach to 1011 in the colon, up to 1 million bacteria per cm2 of skin! In total, our entire digestive tract contains 100,000 billion bacteria, and our intestinal microbiota alone weighs nearly 1.5 kg in an adult (Figure 1).
1.1. Bacteria, the major microorganisms of our microbiotas
A microbiota refers to a complex community of microorganisms that can consist of yeasts, archaea, fungi, viruses and bacteria. Bacteria are the most abundant, which is why a shortcut between microbiota and bacteria is often made. To date, these are therefore the best known and described bacteria (especially in the distal parts of the digestive tract, i.e. the colon). For example, yeast from the digestive tract accounts for less than 0.5% of the intestinal microbiota, while bacteria account for more than 90%. All these microorganisms live in community but also interact with our cells: there is a constant and dynamic balance between these populations whose relative abundance can constantly evolve. Some communities will be altered after a mouthwash or antibiotic treatment, others will take advantage of this to occupy the space and build a niche. The population dynamics that form microbiotas result from interactions with the environment. Microbiotas can be described by the micro-organisms that compose them: a “mapping” is then established, at a given time, of the species present and their quantity.

We can access a large majority of our intestinal microbiota through our stools (fecal samples); although they are easy to obtain, the intestinal microbiota has long kept its secrets. The reason is simple: microorganisms, and especially the bacteria that are so numerous in the intestine, are very difficult to grow because they are very sensitive to the presence of oxygen, which kills more than 60% of them. That’s why they like to confine themselves to the lower part of the digestive tract (colon): in this area, no oxygen! These bacteria also have food preferences and nutrient needs that are still poorly understood. If they are unable to obtain large quantities by culture in the laboratory, scientists can decrypt their DNA. Major high-throughput DNA sequencing projects of our microbiotas were launched between 1995 and 2005 [1]. They have made it possible to establish fine maps of the microbiotas hosted on and in our body and the genes they carry (Figure 2). Different molecular biology and sequencing methods have been used to develop “metagenomes” that represent all sequences of all genomes of dominant and sub-dominant bacteria in an ecosystem (see DNA barcodes to characterize biodiversity). Thus, the intestinal metagenome has made it possible to establish a catalogue of bacterial genes representative of individuals according to their state of health, their genetics, their diet and their living conditions.
In addition to the metagenome approach, sequencing can be more partial by targeting the gene encoding the small ribosome subunit (16S) to describe the bacterial species present within the microbiota. This makes it possible to characterize the species and assess their relative quantities.
1.2. The 2000s: conquering human microbiotas
So let’s detail this bacterial world that inhabits us. The intestinal microbiota contains 800 to 1000 species of bacteria, most of which belong to two bacterial groups (or phyla): Firmicutes and Bacteroidetes (see Figure 2). Proteobacteria, Actinobacteria, Verrucomicrobia and Fusobacteria are also present but in the minority (Figure 2). Within these phyla, there are bacterial families, such as the Lactobacillus family of the Firmicutes phylum. In these families, bacterial species, such as Clostridium coccoides, are distinguished. A large fraction of the species is specific to each individual. Archaea [2] are often present in the intestinal microbiota, especially those involved in methane production.
An individual’s microbiota is considered as unique as his or her fingerprints! However, some species are considered as “founders” such as Faecalibacterium prausnitzii or Akkermansia muciniphila : they are present in the majority of individuals and their absence seems to be associated with pathologies.
The DNA of a human cell contains nearly 3 billion bases organized into 25,000 genes. But bacteria only have about one million bases constituting 2000 to 3000 genes. If we compare a human cell to a bacterium, the genetic potential of the bacteria is therefore 10 times lower. However, if we consider the 500 to 1000 species that make up our intestinal microbiota, bacteria can express 100 to 150 times more genes than the host that hosts them.
Launched between 1995 and 2005 (see [1]), the major DNA sequencing projects for our microbiotas have made it possible to draw up detailed maps of their genes. Catalogues have thus been established, grouping 3 to 10 million genes found in the different bacterial species of human gut microbiotas. These genes allow bacteria to perform specific functions (digesting nutrients, producing vitamins…) and are necessary for the proper functioning of the human being. Thus, the more varied the genetic heritage carried by microorganisms, the greater the metabolic potential of the microbiota. Between different individuals, there is a diversity of species that make up the intestinal microbiota, but the functions performed by bacteria can be common or redundant.
These catalogues of bacterial genes in the microbiota make it possible to compare individuals, or groups of individuals, according to their age, living conditions and health status. The diversity of genes in the intestinal microbiome could even be a marker of susceptibility to certain diseases: the more different bacterial genes you carry in the intestine, the more beneficial this gene capital seems to be for your health.
1.3. Human “oral”, “vaginal” and “pulmonary” microbiotas
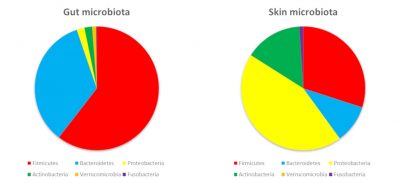
The surfaces of our body are colonized by bacteria belonging to the same phyla as those in our digestive tract. On the other hand, the abundance of phyla will be different. Main explanation? Nutrients provided by the skin and local conditions (oxygen, acidity…) promote the growth of some bacteria rather than others (Figure 3).
The microbiota of the skin is mainly composed of Firmicutes, Proteobacteria and Actinobacteria… including a famous propionibacteria: Propionibacterium acnes, the acne pimple bacteria! The vaginal cavity contains mainly Firmicutes with a high prevalence of Lactobacilli (up to 70% in women). Indeed, these bacteria resist the acidity of the vagina (pH 4.5 in women): they even help to maintain this acidity and are also resistant to oxygen. If the vaginal ecological niche is therefore favourable to the predominance of Lactobacilli, they represent less than 1% of the intestinal microbiota.
The pulmonary microbiota was described in 2010. It has long been known that pathogens can create a niche in the lungs and induce respiratory disease. But it was not until current sequencing methods were used to better understand the bacteria naturally present in a healthy individual’s lung. This pulmonary microbiota contains about 10,000 bacteria per gram of lung or fluid in the bronchi and alveoli of the lungs. It is mainly composed of Proteobacteria, Firmicutes and Bacterides. A new area of research is opening up to better understand the contribution of bacteria to lung immunity and the susceptibility of individuals to certain respiratory diseases.
As true bacterial ecosystems, our various microbiotas are therefore highly dependent on the territories they colonize and sensitive to all external interventions. This is why the composition of microbiotas in the human body is not fixed in time; the ecosystems they constitute are dynamic and evolve according to selection pressures. For example, menstruation disrupts the balance between different lactobacilli in the vagina. Intensive use of soap can trigger a death toll of bacterial species, promoting the proliferation of yeasts and causing the appearance of a yeast infection of the genus Candida. Food and more broadly all the products we consume such as drugs (antibiotics, antacids…) modify bacteria in the oral cavity and intestine.
2. Focus on the intestinal ecosystem
Biological activities that preserve homeostasisA set of biological regulations that will maintain a balance, such as the regulation of our body temperature. and physiology result from the activities of human cells, microbiotas and their constant and mutual interactions. For example, the functions of the digestive tract (digestion, protection against pathogens) are provided by our cells but also by bacteria. The intestinal microbiota thus ensures a large number of biological functions that contribute to our health. It cooperates with our own cells to ensure the digestion of many foods, protection from infection and the maturation of our acquired and innate immune system. The intestinal microbiota can also contribute to extra-intestinal functions by producing molecules that will induce a biological reaction and can act remotely, including on the brain.
2.1. The birth of an ecosystem
Bacteria and our intestinal cells, forming the digestive tract, exchange signals, tolerate each other and sometimes compete for nutrients. The digestive tract must therefore be considered as an ecosystem; its functions and structure depend on the interactions between bacteria and cells in the surrounding environment and on the flow of matter and energy.
The dynamic balance between bacterial and cellular populations in the intestine is co-constructed at birth. This construction of the intestinal ecosystem is progressive and sequential. At birth, our body can be considered free of microorganisms: colonization begins as soon as contact with the environment begins. Bacteria of the Lactobacillus (Enterococcus) and Enterobacteriaceae (E. coli) families as well as Bifidobacteria colonize the digestive tract from birth. Called “primo-colonizers”, the first bacteria reduce the oxygen level, which will then favour the arrival of bacteria too sensitive to oxygen such as Clostridium leptum. These primo-colonizing bacteria are abundant and dominant in the perinatal period, then become subdominant after 2-3 years. This process of primary colonization depends on many parameters, including birth conditions and the baby’s feeding habits. For example, bacteria in breast milk contribute to the diversification of the child’s intestinal microbiota. It has also been found that intestinal microbiotas differ between children born by cesarean section or vaginal route. In the first case, the microbiota of children has a composition close to the bacterial populations of the environment (hands of medical staff, skin). While vaginally born children have an intestinal microbiota closer to that of the mother’s vagina. Primo-colonizing bacteria play a major role for the newborn since they establish the first dialogue with the host. The acquisition of the microbiota at birth can then impact the implantation of other bacteria.
During the first three years of life, the intestinal microbiota is formed following primary colonization, then according to the diet and bacteria encountered in the food, the environment and the people around the child. However, our current lifestyle would reduce exposure to bacteria too much. The cause: too wide use of antibiotics, and too sanitized living environments. This could delay or compromise the establishment of the symbiosis between the host and its microbiota. The alteration of this symbiosis could in turn lead to defects in the maturation of the immune system. At the end of the day: an increased risk of diseases such as allergies, including allergic asthma or inflammatory diseases.
2.2. Bacterial populations of the microbiota: a state of equilibrium
We have already supported the flexibility of the intestinal microbiota with many examples. The balances between populations of microorganisms always oscillate according to living conditions and diet. Microbial population dynamics are also shaped by microorganisms among themselves, which can be “caregivers or helpers”, competitors or pathogens. Thus, the microorganisms brought by fermented foods contribute temporarily to our intestinal microbiota. When you eat a 100 g yogurt, you ingest about a billion bacteria belonging to two species: Lactobacillus bulgaricus and Streptococcus thermophilus. Even if these microorganisms brought by food do not create a permanent niche, their passage modifies the composition and activity of our microbiota. For example, by producing lactate, lactic acid produced by food-borne bacteria may favour lactate-consuming bacteria.
The balance of the microbiota can also be very disturbed. When this goes until the existing balance is broken, it is called “dysbiosis“. Significant dysbiosis appears, for example, after antibiotic therapy or a sudden change in diet. In everyday life, we know that a change of diet can lead to diarrhea, a visible manifestation of a severe disruption of the intestinal microbiota. In the majority of cases, microbiota changes are transient and related to a particular and specific event. This is why the microbiota is considered resilient to moderate stress. This implies that the intestinal microbiota finds a structure close to the one it had before stress.
But there are also situations where the composition of the microbiota will be permanently disrupted. In this case, the mutual benefit that usually occurs between the microbiota and its host is no longer preserved. Everything happens as if they no longer adapt to each other. Faced with a modified microbiota, the response of intestinal cells is inadequate. Sometimes this leads to a host pair/explosive microbiota causing alterations in the body. Dysbiosis is a stage at which the microbiota and host are no longer in symbiosis. It is associated with states of fragility or proven pathology. For example, in patients with chronic inflammatory disease, the composition of the intestinal microbiota is characterized by an overabundance of enterobacteriaceae (E. coli) and a decrease in founder bacteria such as F. prausnitzii. In these patients, this dysbiosis is accompanied by inflammation of the intestinal mucosa and alteration of the intestinal barrier, which are involved in the pathology.
2.3. Human cells and bacteria participate in digestion
Food is degraded by the enzymes of our cells: lipases, amylase, saccharases… Enough to allow us to assimilate fat, digest proteins or starch. However, we do not have the enzymatic equipment to digest all our food.
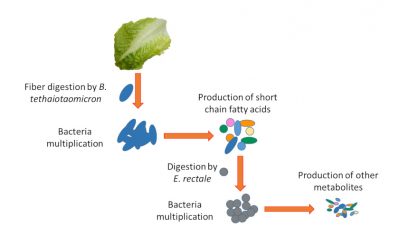
For example, our cells do not digest plant fibers that are composed of oligopolysaccharides. On the other hand, some bacteria are armed with an enzyme arsenal capable of digesting these fibres (e.g. Bacteroides thetaiotaomicron). They transform these fibres made of complex sugars into simpler nutrients that can be used by our body. But other bacteria are like our cells that are unable to digest fibre and therefore depend on the simple nutrients produced by their counterparts (e.g. Enterococcus rectale). At this stage, we can see the complexity of the ecosystem. If the fibres are no longer digested because they are not in the diet or because bacteria such as B. thetaiotaomicron digesting them are altered, the survival of bacteria such as E. rectale will be compromised (Figure 4).
Once degraded, the nutrients are transported into the intestinal cells by proteins present in the membrane of the cells called “transporters”. From the intestinal cells, these nutrients are exported into the bloodstream by other transporters. The presence of these carriers, essential for the passage of nutrients through our body, is controlled by both bacteria and human cells. For example, the installation of iron transporters in intestinal cells depends in part on intestinal bacteria. Any modification of bacteria can therefore cause disruptions, not only to digestion but also to the passage of nutrients through the body.
Finally, bacteria don’t just help us digesting. They also produce nutrients, including vitamins such as vitamin K, which is essential for blood coagulation and without which we would suffer from bleeding.
2.4. A microbiota/intestinal ecosystem: an essential barrier against intruders
Located at the interface between the outside world and our inner environment, the digestive tract plays an essential role as a barrier. Again, our cells and the microorganisms we host cooperate to protect us. Bacteria shield against intruders and shape our immune system, while our cells form a selective cellular and immune barrier.
One of the major roles of the intestinal microbiota is to prevent pathogenic microorganisms from proliferating. Bacterial density is therefore essential to maintain this barrier. One of the most well-known conditions where this balance is disrupted is antibiotic therapy. Antibiotics not only eliminate the bacteria responsible for the infection, but also all intestinal bacteria that are sensitive to the antibiotic. The intestinal microbiota will therefore be all the more altered if the antibiotic is targeting a broad-spectrum bacteria, i.e. capable of killing bacteria from very different families. This hecatomb allows pathogenic bacteria to proliferate. Clostridium difficile infection is one of the possible complications of antibiotic therapy. Thus, the longer and broader the antibiotic therapy, the greater the risk of C. difficile infections.
The intestinal epithelium is the first layer of cells that lies at the interface between the light of the intestine (where the microbiota is confined) and the host’s internal environment. In constant renewal, this epithelium is made up of proliferating stem cells and differentiated cells that participate in the absorption of nutrients or the secretion of molecules. The balance between proliferation and differentiation is essential to ensure effective protection. Hyper-proliferation of the epithelium is a defence that can help to drive out pathogens. Dysbiosis is observed when the epithelial barrier appears looser and less effective at filtering bacteria or bacterial fragments.
The intestinal epithelium also plays an essential protective role through the production of mucus. By lining the epithelium, mucus is similar to a viscous gel made up of complex glycoproteinsProteins on which sugars are added, which give them specific biological properties. Present, among other things, on cell membranes; they promote interactions between cells.. It is produced by specialized cells of the intestinal epithelium: mucus producing and secreting cells. This mucus keeps bacteria away from the epithelium, preventing their direct interaction with the cells. The barrier provided by mucus can be modulated by food and by the bacteria themselves. Bacteria capable of digesting dietary fibre are also fond of the complex sugars in mucus. The latter is therefore also a source of nutrients for certain bacteria in the microbiota. In case of a diet low in complex sugars, B. thetaiotaomicron will degrade the mucus; but as compensation, it can produce signals stimulating mucus production. A good example of the mutualism of the microbiota/intestinal ecosystem!
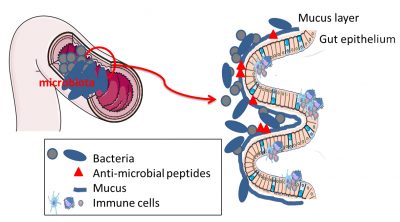
In addition to the physical distance imposed by mucus, there is also a chemical barrier. Some intestinal cells produce metabolites with anti-microbial actions. These antimicrobial compounds will keep bacteria away from intestinal cells, avoiding any intrusion or uncontrolled contact (Figure 5).
2.5. Bacteria that educate our immune system
Our immune system is there to fight infections whether they are viral, fungal or bacterial. But it also helps to control intestinal bacteria. This mechanism involves the production of specific antibodies. These antibodies of type IgAAbbreviation for Immunoglobulin A, one of the forms (or isoforms) of immunoglobulins (also called antibodies). Immunoglobulins are proteins produced by cells of our immunity, the B lymphocytes, in response to a foreign molecule, which may be a bacterium. The different isoforms (A, G, M…) are distinguished from each other according to their role. are discharged into the digestive tract: they trap bacteria and thus contribute to their elimination in the faeces.
Our body is well equipped to fight the invasion of bacteria, while tolerating their presence. Among this equipment, our cells have bacteria sensors more precisely, some components of these bacteria. These detectors, called receptors, will recognize bacterial patterns such as lipopolysaccharidesmolecules with a lipid and a carbohydrate portion. (LPS) that differ according to the bacteria [3]. When these LPS are detected, they trigger a chain reaction that activates the immune system to control the infection. The bacteria in our intestinal microbiota are no exception to this rule: they too have LPS. On the other hand, if our detector judges that the detected LPS does not require an immune response, the LPS-producing bacteria are not eliminated. This tolerance towards our intestinal bacteria is therefore a fragile balance; any disruption of the microbiota can lead to deregulation and abnormal activation of the immune system.
Bacteria also play a crucial role in educating our immune system. Indeed, in “axenic or germ free” animals (without microbiotas and therefore entirely sterile), the immune system is immature. This proves that our own microbiota educates our immunity. The successive implantation of different bacterial species from microbiota to early childhood is crucial to allow this maturation of the immune system. Thus, disrupting this sequence of acquisition of bacterial species seems likely to modify this immune maturation and contribute to the development of certain allergies.
Ultimately, our body must therefore contain the bacteria in the digestive tract and these bacteria must make themselves tolerable. However, these bacteria are also essential to teach us how to fight pathogenic bacteria.
2.6. The microbiota/intestinal cells couple: a dialogue that can be heard throughout the body
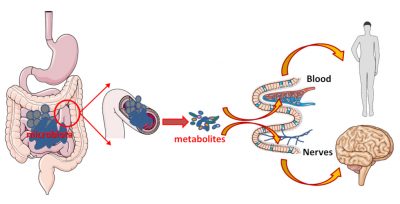
The microbiota/intestinal cells pair is effective in digesting, absorbing and providing us with nutrients. But this inseparable couple also produces signals that can spread throughout the body and have remote effects. These signals can be sent by bacteria, by their metabolites, or be fragments of bacteria. These signals can be transported via the intestinal cells to the blood to act on our organs. But these metabolites can also act on site. In this case, the intestine, very rich in nerve connections, will serve as an intermediary: the metabolites produced by the bacteria activate these nerve connections and thus transmit the information (Figure 6).
Physically confined to the digestive tract, the microbiota/intestinal ecosystem is thus able to exert its effects not only locally, but also remotely via metabolites and neural networks and/or thevague nerveAlso called pneumogastric nerve. There are two, each innervating one side of the body. These nerves start from the spinal bulb, the top of the spinal cord, and innervate the heart, lungs and intestine. The information transmitted is unconsciously (autonomously).. Hence the idea that the intestinal microbiota would play the role of conductor in what is defined as the intestinal/brain axis.
3. Disruptions in the intestinal ecosystem: causes of the development of diseases
The dialogue and symbiosis between the microbiota and the host is fragile: any change in the intestinal bacterial composition or host can disrupt the ecosystem. In recent years, thanks to the high throughput sequencing performance, research has shown that many diseases are associated with dysbiosis: chronic intestinal inflammation, obesity, liver inflammation (non-viral hepatitis)… But dysbioses are also associated with cardiovascular diseases, allergies and behavioural or psychiatric diseases (stress, depression, autism).
One of the major current issues is therefore to clarify whether dysbiosis is a consequence of these various diseases or one of the causes. The causal role of the intestinal microbiota as a cofactor in the development of many diseases is already recognized; this is the case in chronic inflammatory bowel diseases, certain liver diseases, cardiovascular diseases, certain allergies and even neurological diseases such as autism.
3.1. Microbiota and digestive diseases
The first pathologies where the causal role of the intestinal microbiota has been questioned are digestive diseases. Some bacteria, such as F. prausnitzii, appear to play an important role in chronic inflammatory diseases of the digestive tract, particularly in Crohn’s disease. Thus, the most serious or relapsing patients have the lowest levels of F. prausnitzii. This bacterium produces metabolites that moderate inflammation and thus reduce seizures. Current research is therefore aimed at cultivating this bacterium and deciphering its mechanisms of action. However, this highly oxygen-sensitive bacterium poses a real technical challenge to researchers who are struggling with the possibility of producing it on a large scale in order to develop new treatments.
3.2. Microbiota and metabolic syndrome
The metabolic syndrome is a set of metabolic alterations associated with overweight or obesity. It causes various complications, including cardiovascular disease and diabetes. Complications associated with obesity are linked to an alteration of the intestinal barrier and a decrease in bacterial diversity. In addition, regardless of the treatment, symptom improvement is systematically associated with greater bacterial diversity and improved intestinal barrier.
Microbiota and obesity. The increase in the number of overweight or obese individuals in the world is due to overeating but also to a change in the nutritional quality of food. Fibre made up nearly 60% of our diet just a few hundred years ago: it now accounts for only about 10% of our daily intake. However, these changes do not always explain why some individuals will develop higher obesity or associated complications (cardiovascular disease, type 2 diabetesCharacterized by chronic hyperglycemia, i.e., blood glucose levels are too high. The insulin that regulates this level of glucose in the blood is still produced but it can no longer act on the organs, we talk about insulin resistance.). Given the different functions performed by the intestinal microbiota, particularly metabolic, its role in obesity is widely studied.
Obesity is associated with a decrease in the diversity of bacterial species and therefore of bacterial genes present in the intestinal microbiota. The microbiota of an obese patient can make mice obese when transplanted. Moreover, in obese individuals, a slimming diet will be all the more effective if its bacterial diversity is high. It is therefore now obvious that the microbiota plays a role in weight gain. On the other hand, if the presence of intestinal bacteria A. muciniphila and F. prausnitzii appear to be associated with a beneficial profile of the intestinal microbiota, they cannot counteract dysbiosis on their own.
Cardiovascular diseases and type 2 diabetes. Complications frequently associated with obesity include cardiovascular disease and type 2 diabetes. However, not all overweight or obese individuals develop these complications. In addition to their genetic heritage, the intestinal microbiota contributes to individual susceptibility to these complications.
Changes in the bacterial composition of the intestinal microbiota have been observed in patients with type 2 diabetes. Some species are particularly decreased in these patients. Among them: F. prausnitzii and bacteria of the genus Roseburia. Akkermansia muciniphila is also decreased; and as in obesity, its administration to rodents clearly reduces insulin resistance. But the interactions between the metabolites produced by the intestinal microbiota and the functions of our body are just beginning to be deciphered, suggesting the complexity of the influence of the host/bacteria ecosystem for certain diseases.
Among the complications of the metabolic syndrome, cardiovascular diseases remain to this day the leading cause of death in France. In these pathologies, atheroma plaquesPlates composed of various components (cholesterol, cellular debris and calcium) that will settle on the artery and contribute to its clogging. When the plaque clogs the vessel, it is called thrombosis. Atheroma is the leading cause of most cardiovascular diseases. can rupture and form clots blocking blood vessels. However, intestinal bacteria play a role in the formation of these plaques. Indeed, according to the bacteria housed in our digestive tract, certain food compounds lead to the formation of molecules that promote atheroma plaque (TMATrimethylamine then transformed by the liver into TMAOTrimethylamine oxide). Thus, the consumption of meat products containing cholineNutrient that will be incorporated into different biological molecules present in our body. Among them, lecithin is a phospholipid that contains choline., lecithin or carnitineNon essential amino acid. favors this production. Bacteria have also been found directly in these plaques, whose origin seems more buccal than intestinal.
While the high-fibre Mediterranean diet has a beneficial effect on cardiovascular risk, no bacteria involved in this beneficial effect have yet been identified. Used as probiotics in rodent studies, some Lactobacilli have also shown some beneficial effects on cardiac function. In the end, if the role of the intestinal microbiota seems to be proven in the development of cardiovascular complications, treatments acting on bacteria have yet to be developed.
3.4. Microbiota and neurological diseases
Communication between our intestinal bacteria and our brain involves an extremely wide variety of metabolites that they can produce. As described in Chapter 2.6, these metabolites play a role in our neurons, either through the blood or by acting directly on nerve endings in our digestive tract. In addition, it has been shown that the nature of our intestinal microbiota can affect the tightness of the blood-brain barrier; this barrier protects our brain by limiting the passage of many substances that could have a negative effect on its functioning.
The latest advances have shown that the nature of intestinal bacteria has an effect on the state of anxiety and stress but also during depression. In rodents, supplementation with lactobacillus or bifidobacteria (especially B. infantis) seems to improve depressive symptoms. While low levels of some bacteria are observed during depression (e.g., F. prausnitzii), it is not yet known whether this decrease is directly responsible for depressive symptoms.
Dysbiosis associated with certain neurodegenerative diseases has also been observed: Parkinson’s disease, Alzheimer’s disease and multiple sclerosis. However, it is not yet known to what extent this dysbiosis accompanies and contributes to these diseases.
4. The microbiota, a therapeutic target of the future
Restoring the balance of the intestinal/microbiota ecosystem is therefore a real challenge for developing new treatments. In the meantime, food is already a tremendous lever to influence our microbiota. For example, adequate fibres intake in our diet helps to promote the growth of a large number of bacterial species. We could also bring missing or deficient bacteria in certain pathologies, through fermented foods or food supplements rich in bacteria. Among the fibres, some have already demonstrated their beneficial actions on the host by modulating the intestinal microbiota: these are prebiotics.
Intestinal bacteria with beneficial effects on our health could be consumed as food supplements. This makes up for their absence or decline observed in certain diseases. For example, the bacterium F. prausnitzii is dominant in a healthy individual… but is weakly present in many diseases, including chronic inflammatory bowel diseases (IBD).
The use of the intestinal microbiota in care protocols has already become standard practice in hospitals. Microbiota transfers are prescribed, for example, to treat recurrent C. difficile infections that are resistant to conventional antibiotic treatments. In practice, this involves transferring faeces from a healthy donor to re-seed the recipient’s intestine. After this faecal transfer, patients no longer suffer from their C. difficile infection. The microbiota ecosystem is therefore already being used as a therapeutic strategy. The use of this faecal transfer technique will probably be extended to other pathological situations.
These are all therapeutic strategies based on the restoration of a balance of the intestinal/microbiota ecosystem essential to the proper functioning of our body [4].
Cover image. Painting by Teppei Ikehila: Fascinated by the magic of bacteria, small cells capable of infinite reproduction, extraordinary shapes and colours, Teppei Ikehila makes it a privileged inspiring theme.
[1] See for example: Human Microbiome Project (http://hmpdacc.org/), Metahit (http://www.metahit.eu/)
[2] In ruminants, archaea can represent 0.3 to 3.3% of bacteria in the stomach or rumen. In humans, the presence of arches within the intestinal microbiota does not seem to be systematic. However, there is probably a bias in that many studies do not include in their study.
[3] These receptors are “Toll-like” or TLR receptors when they are present at the cell membrane. They can also be present in cells and recognize bacterial patterns of peptidoglycan types. These are NOD receivers.
[4] Kapel et al (2014) Practical implementation of fecal transplantation. Clin Microbiol Infect. 20(11):1098-1105; French Group of Faecal microbiota Transplantation (FGFT) (2016) Faecal microbiota transplantation in recurrent Clostridium difficile infection: Recommendations from the French Group of Faecal microbiota Transplantation. Sokol Dig Liver Dis. 48(3):242-247.
The Encyclopedia of the Environment by the Association des Encyclopédies de l'Environnement et de l'Énergie (www.a3e.fr), contractually linked to the University of Grenoble Alpes and Grenoble INP, and sponsored by the French Academy of Sciences.
To cite this article: CASSARD Anne-Marie, THOMAS Muriel (May 7, 2019), Human microbiotas: allies for our health, Encyclopedia of the Environment, Accessed July 27, 2024 [online ISSN 2555-0950] url : https://www.encyclopedie-environnement.org/en/health/human-microbiotas-allies-for-our-health/.
The articles in the Encyclopedia of the Environment are made available under the terms of the Creative Commons BY-NC-SA license, which authorizes reproduction subject to: citing the source, not making commercial use of them, sharing identical initial conditions, reproducing at each reuse or distribution the mention of this Creative Commons BY-NC-SA license.