Tropical Cyclones: development and organization
PDF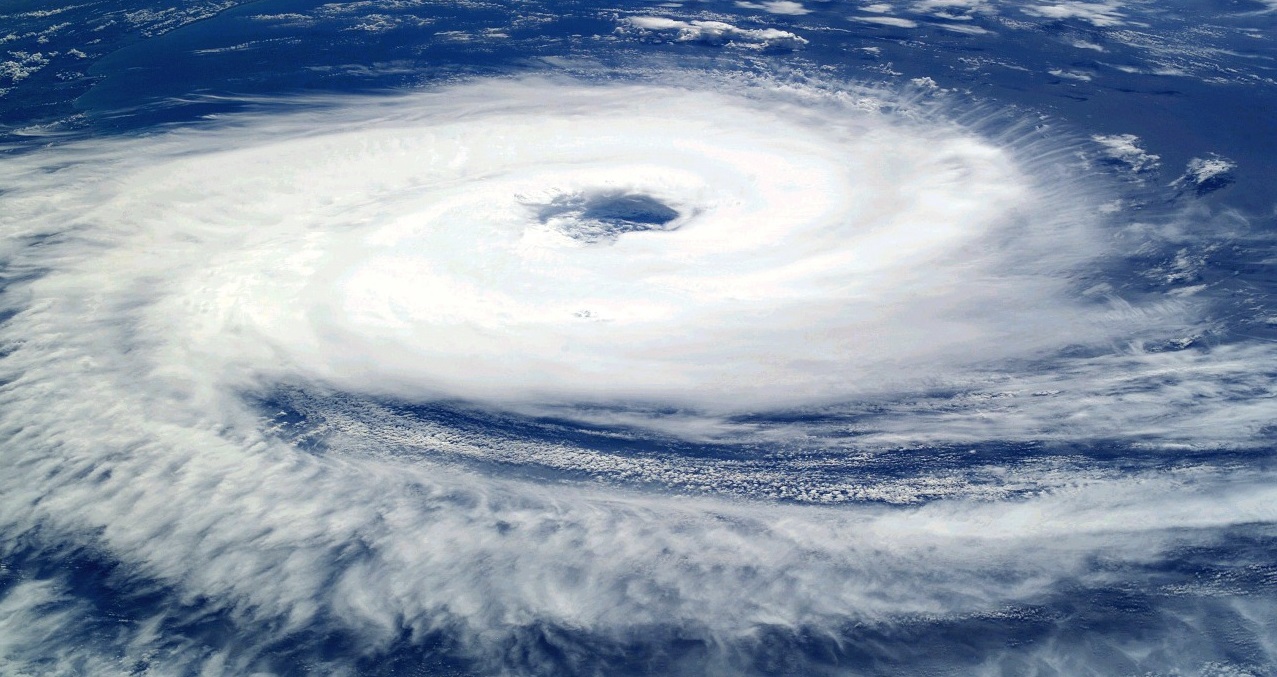
Tropical cyclones extract their energy from the heat stored in the tropical oceans and transform it into fierce winds, devastating rains, monstrous waves that devastate the lands they approach. Their organization, highlighted in the above photograph taken from the International Space Station (Hurricane Katrina, August 2005), is influenced by an interplay between dynamics and thermodynamics.
1. Tropical cyclone definitions and climatology

According to the definition of the World Meteorological Organization (WMO), tropical cyclones are “atmospheric disturbances of a few hundred kilometres wide scale, originating over tropical or sub-tropical waters and presenting an organized thunderstorm activity and a cyclonic circulation [1], more intense at the surface than at altitude“. These phenomena have different names depending on their intensity, measured by the strength of the surface wind. A “Tropical Disturbance is a persistent thunderstorm region with moderate winds that outline an overall rotation. A “Tropical Depression” is characterized by closed circulation and winds blowing at less than 17 m s-1 (about 60 km/h). Wind speed can reach 32 m s-1 (about 120 km/h) in a “Tropical Storm”. Beyond that, it is a “Tropical Cyclone”, also called a “Hurricane” on the Atlantic and northeast Pacific, a “Typhoon” on the northwest Pacific. Cyclones are classified according to the maximum wind speed or, equivalently, the minimum surface pressure. The scale proposed in 1977 by the Americans H.S. Saffir and R.H. Simpson has 5 levels.
Each year, 80 to 90 tropical storms occur during the summer and early fall, about half of which develop into cyclones. The northern hemisphere is by far the largest, due to the absence of cyclones over the South Atlantic and South-East Pacific, where the ocean is not warm enough. The Pacific Northwest with 25 depressions and storms, and 15 typhoons, accounts for nearly a third of the world total. It is the only basin where cyclones are observed all year round, with a maximum in summer and autumn. Over the northeast Pacific, there are an average of 15 depressions and storms, and about ten hurricanes. The North Atlantic generates an average of 10 depressions and storms, resulting in about 5 hurricanes per year. The northern Indian Ocean accounts for only 5% of the world total, but due to the shallow depth of the Bay of Bengal, low coastal elevations and high population densities, they often cause considerable damage. Their distribution shows a first maximum in May – June and another secondary in October – November. Between these two periods, the Indian monsoon generates strong upper winds, which are unfavourable to the development of cyclones. In the southern hemisphere, 10 depressions and storms, 5 cyclones are observed during the southern summer and autumn over the southwestern Indian Ocean. The southeast of this ocean and northern Australia produce an average of 7 depressions and storms, and 3 cyclones per year. Finally, 10 depressions and storms, and 5 cyclones occur annually over the southwest Pacific. Interannual variability is quite high in each basin, but fluctuations in opposite directions between different oceans generally compensate for local variations.
2. Formation of tropical cyclones
The development of a tropical cyclone disturbance requires specific conditions:
- The ocean surface temperature must be above 26°C with a relatively homogeneous layer at least 60 metres deep, because the heat and especially the humidity that the air takes from the ocean are the “fuel” of the cyclone machine.
- High atmospheric humidity minimizes the evaporation of precipitation into dry air and the formation of cold downdrafts.
- A low wind shear [2] prevents winds of too different forces or directions depending on altitude from distorting the cyclonic vortex and blocking its evolution.
- On a large scale, the airflow converges in the lower layers and diverges at high altitudes to promote thunderstorm development.
- Large-scale cyclonic circulation facilitates the organization of the storm cluster.
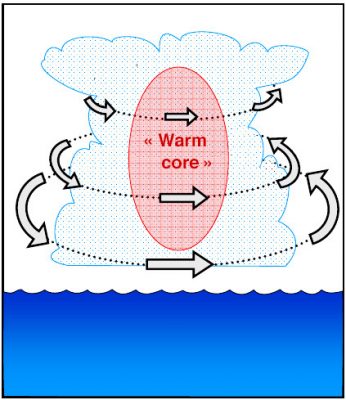
Above 5 to 10° latitude, the Coriolis force [3] due to the Earth’s rotation has a sufficient amplitude for the “thermal wind balance” to require that a persistent warm anomaly at altitude be accompanied by a cyclonic wind rotation whose intensity decreases with altitude. Thus, in response to the heat released by the condensation of water vapour within the clouds of the disturbed area, the wind forms cyclonic eddies of varying intensity, a few dozen to a few hundred kilometres wide. Such circulations may persist long after the deep convective clouds that produced them have dissipated. They also facilitate new stormy developments that can strengthen them. At the same time, the colder air pockets brought by downdrafts into the lower layers of the atmosphere must disappear. The transfer of heat and humidity from the ocean to the atmosphere restores energy to this fresh air. After some time, it becomes warm and humid enough to generate thunderstorm activity again. Gradually, the atmosphere warms and humidifies. The formation of downdrafts by cooling due to evaporation becomes more difficult and they are displaced into the periphery of the storm cluster, a few hundred kilometres away.
As thunderstorm cells develop, a large warm anomaly forms at altitude in the central part of the cluster. As the heated air column is lighter, the surface pressure gradually decreases. The force that attracts air to the low pressure centre is balanced by the Coriolis force and by the centrifugal acceleration of an overall cyclonic movement, reinforced by the aggregation of the various successively created eddies. Dragged into this movement, the cloudy bands wind in wide spirals. The wind blows increasingly strong, collecting moisture and heat from the ocean surface, which feeds the storm updrafts, warms the atmosphere, reduces surface pressure and intensifies the vortex. When cyclonic circulation is sufficiently developed with a strong surface depression, a weak downdraft is established at the centre of the disturbance. This movement dries the air, the cloud mass dissipates locally and the “Eye” of the cyclone appears.
3. Energy cycle and maximum intensity
A mature tropical cyclone is a thermal engine whose heat source is the condensation of water vapour. This is not the result, as has long been believed, of the presence of large-scale thunderstorm instability. The tropical oceanic atmosphere is generally close to neutrality, which does not allow the development of large-scale upward movements. A very large part of the energy of cyclones comes from evaporation at the ocean surface, forced by winds of increasing intensity.
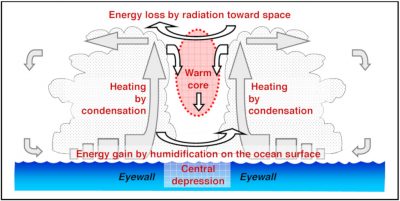
Carried by powerful updrafts to an altitude of about 15 kilometres, the cloudy air moves away from the centre of the cyclone in a divergent and anticyclonic movement, and loses energy by thermal radiation towards space. The return to the surface is accomplished with the downward flow that predominates at large distance. In the eye, the slight downward movement of the air induces a heating that strengthens the central depression, attracts outside air from the lower layers and ensures that the cycle is maintained. This diagram represents an ideal thermodynamic cycle “of Carnot” and makes it possible to estimate a “Maximum Potential Intensity”, minimum pressure or maximum wind, that a cyclone can reach in an environment characterized by its latitude, the temperature of the ocean and that of the tropopause at about 15 kilometers of altitude. The values obtained are in good agreement with the extremes observed, showing that this cycle represents an energy optimum for tropical cyclones.
4. Maturity structure
But few cyclones reach their maximum potential intensity because the details of internal circulation are more complex than the previous diagram, and the efficiency of energy conversions is rarely optimal.
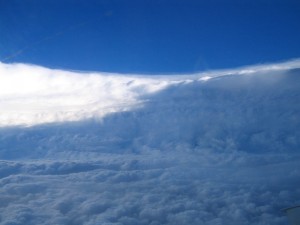
In the “Eyewall” of cumulonimbus clouds, which surrounds the eye a few tens of kilometres from the circulation centre, there are powerful vertical motions with the highest precipitation, organised in narrow spiral bands or rings, and the strongest winds frequently reach 200 km/h, sometimes exceeding 300 km/h. The wind speed is maximum at an altitude of a few hundred meters. Further down, friction on the ocean surface slows it down. This characteristic of tropical cyclones differentiates them from disturbances in mid-latitudes (where the strongest winds are at high altitudes) and makes them much more devastating at comparable intensities.
The “Core” is the region, a few hundred kilometres wide, where the air follows closed trajectories around the low pressure centre. The cyclonic “Primary Circulation” that circulates around the eye is much more intense than the radial (which goes from the outside to the inside, and vice versa) and vertical “Secondary Circulation”. Near the surface, the wind is slowed by friction on the ocean and tangential acceleration cannot compensate for the force that attracts air to the central depression. The resulting radial acceleration produces a converging flow in the lower layers, directed towards the centre of the cyclone, which feeds the rising currents.
In the peripheral zone, hundreds of kilometres beyond the core, the horizontal flow is less symmetrical and the radial component of the wind is proportionally larger. Long bands of precipitation, locally intense and a few tens of kilometres wide, extend mainly eastward in the convergence zone between cyclonic circulation and the easterly trade winds that prevail in the tropics. The southeast quarter is favoured in the northern hemisphere, the northeast one in the southern hemisphere.
5. Changes in structure
The coupling between primary and secondary circulations implies that the Eyewall cannot be stable. The upward movement induces a compensating downward movement in the Eye and an acceleration of the surface wind at a distance a little closer to the circulation centre. The stronger wind at this location increases surface friction and moisture input, which shifts the Eyewall inward. The result is a tendency for the Eyewall to gradually shrink. Outside, the shrinking of peripheral rainbands also generates upper-level downward motion in their inner side that gradually chokes the Wall. This effect leads to a temporary decrease in the intensity of the cyclone when the Eyewall progressively dissipates, before a new intensification when the external bands have gradually organized themselves into a new Eyewall.
Above 30 degrees latitude on average, cyclones weaken when they arrive on continents or over waters that are no longer warm enough to sustain the energy cycle, or by suffering the effects of wind shear – stronger at mid-latitudes – which distorts their vertical structure. Under these conditions, small or low-intensity cyclones dissipate fairly quickly, but the strongest and most extensive ones maintain their organization and intensity, sometimes for several days. A few tropical cyclones interact with the westerly circulation and evolve into a mid-latitude (baroclinic) storm. The probability of such transitions varies according to the configuration of the ocean basin: non-existent over the northeast Pacific or northern Indian Ocean, rare over the southwest Pacific or southeast Indian Ocean, it affects about one in five cyclones over the southwest Indian Ocean, one in four over the northwest Pacific, one in three over the north Atlantic. In early autumn, old Caribbean hurricanes may turn into mid-latitude storms on the west coast of Europe a few days later.
Other changes also occur when a cyclone interacts with relief, when passing over fairly large mountainous islands, such as Luzon in the Philippines, Taiwan, Hispaniola in the Caribbean, or on a coastal barrier such as the Central American Cordillera. The forced lifting of hot and humid air on the windward side of the mountains causes a local reinforcement of the sometimes very heavy rains, leading to floods and landslides with often dramatic consequences. The change in the distribution of storm activity temporarily disrupts the cyclone’s dynamics. Sometimes, the eye disappears as it approaches the island, and reappears a few hours later, several tens of kilometres in the wake of the obstacle.
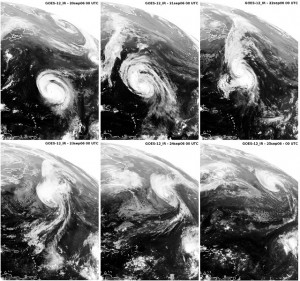

References and notes
[1] Clockwise rotation movement in the southern hemisphere, counter-clockwise in the northern hemisphere.
[2] Vertical variation in wind speed or direction.
[3] “Pseudo-force” directed perpendicular to the direction of movement of a moving body in a uniformly rotating environment.
The Encyclopedia of the Environment by the Association des Encyclopédies de l'Environnement et de l'Énergie (www.a3e.fr), contractually linked to the University of Grenoble Alpes and Grenoble INP, and sponsored by the French Academy of Sciences.
To cite this article: ROUX Frank (July 16, 2019), Tropical Cyclones: development and organization, Encyclopedia of the Environment, Accessed July 27, 2024 [online ISSN 2555-0950] url : https://www.encyclopedie-environnement.org/en/air-en/tropical-cyclones-development-and-organization/.
The articles in the Encyclopedia of the Environment are made available under the terms of the Creative Commons BY-NC-SA license, which authorizes reproduction subject to: citing the source, not making commercial use of them, sharing identical initial conditions, reproducing at each reuse or distribution the mention of this Creative Commons BY-NC-SA license.