Soil biodiversity
PDF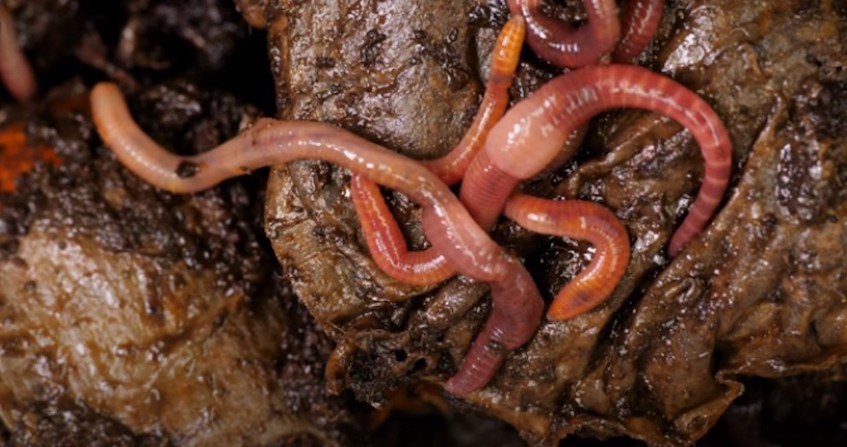
Soil, a major compartment of terrestrial ecosystems, represents a very particular ecosystem since it constitutes one of the most important reservoirs of biodiversity on the planet. Bacteria, fungi, springtails, insects, myriapods and many other organisms live and interact in the soil. This very heterogeneous biological richness is at the very origin of the functioning of the soil and of all the ecosystem services it provides to mankind, such as our food, our living space or our climate. Better characterizing it, better understanding it, can only help us to better manage it in order to safeguard it and perpetuate the treasure it constitutes for future generations.
1. A reservoir of biodiversity
We have all already turned over or seen soil turned over with a spade: we can easily distinguish the life that resides there, at least the visible part (the largest organisms), which is only a small part of the life that the soil harbors. Indeed, most of this diversity of organisms is invisible to our eyes because they are microscopic. The soil is thus one of the main reservoirs of biodiversity, since it hosts about a quarter of the total biodiversity (taxonomic diversity) of the planet [1]. Moreover, the number of “species” is several times higher than those observed on its surface. Biodiversity, a contraction of “biological diversity”, refers to the variety of the living world. It is the diversity of all forms of animal, plant and microscopic life on Earth, and of all the relationships that species (of which we are part) have with each other and with their environment.
Several groups and a great diversity of organisms live in the soil: insects, mollusks, protozoa, algae, bacteria, fungi, etc. Soils have a very high level of heterogeneity, and an extremely large number of habitats, which also contribute to a high level of biodiversity, if the soil is in “good health”. Indeed, the level of biological abundance and diversity can vary widely from one soil to another, depending on a variety of factors, including organic matter content, soil mineral composition (especially texture), pH and soil management practices. This biodiversity is not only very high in number, it can also be functional. Indeed, soil organisms maintain complex relationships with each other, but also influence the entire ecosystem by controlling the circulation of materials essential to plant life (carbon, nitrogen, phosphorus, potassium…) [2].
1.1. Who does what in the soil?
Biodiversity is “the structural and functional variability of the diverse life forms that inhabit the biosphere, at increasing levels of organization and complexity: at the genetic, population, species, community and ecosystem levels” [3] (see What is biodiversity? and Biodiversity is not a luxury but a necessity). This definition highlights the need to understand and measure soil biodiversity in two aspects: the diversity of species and families (taxa) and the diversity of functions.
Taxonomic richness corresponds to the number of taxa (a taxon is a group corresponding to a level of classification of living organisms, such as a species or family) identified in the system under study. For an equal number of individuals, the more taxa present (species for example), the greater the diversity of a community. It is also usual to consider the abundance (number of individuals) of each taxon.
Functional biodiversity describes “who does what in the ecosystem” or “who is what” and not just “who is present” in a given community. Thus, to characterize functional diversity, it is necessary to assign or measure functions (nitrification, degradation of organic compounds…) or roles for each individual in a given community.
1.2. Soil organisms: from smallest to largest
The following sections detail the main groups of microorganisms and soil fauna. It should be noted that viruses are not considered as part of soil biodiversity because, although present in this environment and influencing the population dynamics of organisms, they are not living beings.
2. Soil micro-organisms
Soil micro-organisms are extremely abundant and of great taxonomic and functional diversity. They include bacteria, fungi, microalgae, archaea and protists [5]. The biological functioning of soils is strongly linked to microbial activity, which gives these microorganisms a major role in many soil functions as discussed below.
2.1. Bacteria
Bacteria are by far the most numerous and diverse soil microorganisms [6]. They come in a wide variety of shapes and sizes (mostly smaller than 2 µm). It is estimated that one gram of soil contains about one billion bacteria and between 2000 and 10000 bacterial species depending on their health status [7]. Metagenomic studies (reading DNA barcodes to characterize biodiversity) on different soils (grasslands, forests, arid soils, agricultural soils , etc.), have identified sequences belonging to Proteobacteria (40%), Acidobacteria (20%) and Actinobacteria (13%) [8].
Bacteria play a key role in nutrient recycling, development (through the formation of symbioses), and soil structuring. They also contribute to the regulation of diseases, and to the depollution of contaminated soils. They interact in the rhizosphere of plants (region of the soil directly formed and strongly influenced by the association of roots and soil microorganisms) with which they can form symbioses with plants and they can also be pathogenic, both for animals and plants.
Bacteria play an essential role in the functioning of soils through their mineralization functions (mineralization of organic matter, oxidation-reduction of inorganic compounds, solubilization or precipitation of minerals, transformation of more or less recalcitrant organic compounds …) [9]. They are thus at the base of the regulation of the main biogeochemical cycles of soils (carbon, nitrogen, phosphorus, sulfur…) and they are able to:
- reduce sulfates to sulfites and sulfides (sulfate-reducing bacteria)
- oxidize sulfur (Thiobacillus for example),
- fix atmospheric nitrogen (diazotrophy alone or in symbiosis with plants),
- produce nitrates (nitrifying bacteria),
- make phosphorus available (by alkaline phosphatase for example) [9] etc.
In addition, some bacteria, especially those called PGPR (Plant Growth Promoting Rhizobacteria ), have the ability to develop symbioses with plants, which allow them to increase the availability of nutrients for their growth.
Bacteria can also promote the decontamination of soils contaminated by heavy metals (known as trace metals or TMEs) [10] by modifying their availability, but they are mainly involved in the decontamination of organic compounds such as polycyclic aromatic hydrocarbons (PAHs) by mineralizing them at least partially.
2.2. Fungi
Fungi are commonly classified into two groups: yeasts, which are unicellular, and fungi, which are multicellular and form branches called hyphae. These are the fine white filaments that we often see on the surface of the ground or leaves during a walk in the forest. These filaments constitute a very important biomass (several tons per hectare) and also an impressive network circulating through the soil over long distances: one square meter of meadow or forest soil contains several kilometers of hyphae.
2.2.1. Saprophytic fungi
2.2.2. Mycorrhizal fungi
Mycorrhizal fungi form symbiotic associations with plant roots. These plant-fungus symbioses are extremely widespread and concern 80 to 90% of plant species [16]. In this mutually beneficial relationship, the fungus receives from the plant elements necessary for its growth such as sugars and vitamins. The fungus in turn absorbs various elements from the soil, including phosphorus, which it transfers back to the plant, thus considerably increasing the volume of soil explored by the plants for their growth.
There are two main groups of mycorrhizal fungi: ectomycorrhizal and endomycorrhizal. The ectomycorrhizal fungi form a network of hyphae (Hartig’s network) on the surface of the roots of colonized plants. This forms a peripheral sleeve that serves as an exchange matrix between the plant and the fungus, and the latter is then able to insert itself between the plant cortical cells without being able to penetrate them. The plant hosts of these fungi are mainly of the shrub and tree type. More than 95% of ectomycorrhizae belong to the group of basidiomycetes [17].
AM fungi (exclusively glomeromycetes) are obligate symbiotrophs since without the interaction with the host plant, which provides them with carbonaceous elements, they cannot complete their development cycle. For the plant, the main advantage is a better hydric and mineral nutrition, in particular in phosphorus brought by the fungus, which is most often translated by an increase of the plant biomass compared to a non-colonized plant.
2.2.3. Endophytic fungi
Endophytic (root) fungi have a way of life that is characterized by the colonization of the internal structures of a plant in an asymptomatic way. Indeed, these fungi take nutrients from the host without the latter showing signs of disease and remain entirely in the root tissues, unlike mycorrhizal fungi that colonize plants while developing in the rhizosphere. Endophytic fungi are overwhelmingly derived from ascomycetes and basidiomycetes, of which the three best known members are the DSE (Dark Septate Endophytes), Sebacinales and Trichoderma [19]. Like mycorrhizal fungi, endophytic fungi promote growth and resistance to abiotic stresses in the host plant.
2.2.4. Pathogenic/parasitic fungi
Pathogenic fungi can be primarily parasites of other fungi, animal pathogens or plant pathogens (phytopathogens) [20]. These soil-borne plant pathogenic fungi can infect the roots, or enter its vascular systems and colonize the entire organism by ascending the xylem vessels, although they mainly attack the aerial parts.
3. Soil fauna
If we take the image of observing the soil with a spade, we can easily distinguish animals of very different sizes: this is used to classify the soil fauna into four groups [21]: microfauna, mesofauna, macrofauna and megafauna. The soil fauna is defined as all the animals that have at least part of their life cycle in the soil. This fauna represents nearly a quarter (23%) of the total diversity of living organisms described to date [22] with very heterogeneous horizontal and vertical distributions. Thus, in the brown soils of temperate regions, the fauna is mainly located in the first 10 to 20 cm of the soil and its appendages (litter, dead wood, etc.).
3.1. The microfauna
Invisible to the naked eye, the microfauna is made up of individuals generally smaller than 200µm which can therefore live in the capillary porosity of the soil. Rotifers and tardigrades are part of the soil microfauna, but it is mainly protozoa and nematodes that make up the bulk of this microfauna. Indeed, the protozoa present an abundance varying from 100 to 1000 million individuals per square meter. This group is very diverse as well as their nutrition, but most protozoa can ingest particles and feed on bacteria. These organisms, like nematodes, live in the water film surrounding soil particles [23]. Approximately 30,000 species of nematodes are known to date, but this would only represent about 5% of the number of existing species. They are classified into five trophic groups: bacterivores, fungivores, omnivores (feed on bacteria and fungi), phytoparasites (parasites of plants), and predators (feed on other animals) [23]. Nematodes play a key role in the mineralization and decomposition of organic matter and in the regulation of microbial communities.
3.2. The mesofauna
The mesofauna (or micro-arthropods or meso-invertebrates) consists of animals with a size of about 0.2 to 4 mm. Collembola and mites are the two main representatives of this group. Protoures, diploures, thysanoures, enchytraides, and symphilic myriapods, are also part of the mesofauna but their abundance is much lower.
Collembola are probably the most abundant group of hexapods on Earth (up to 200,000 individuals per m-2 in uncultivated brown soils of temperate regions) and with a high diversity (about 8,000 known species, of which more than 2,000 in Europe (Figure 6). Collembola are essentially decomposers, feeding on mycelial hyphae and organic matter, and are therefore mainly found in soil and litter (dead leaves and wood). They thus play a major role in the decomposition process of the litter by micro-fragmentation and mixing of the organic matter.
Figure 6. Video of springtails on decaying wood. [Source: © Apolline Auclerc with the Direction du numérique de l’Université de Lorraine]
* furca: abdominal appendage that allows the springtail to jump
With more than 48,000 species already described, mites colonize many habitats, especially those rich in organic matter (peat, decaying wood, litter, etc.). Like springtails, most mites contribute to the fragmentation of organic matter by producing fecal pellets and thus indirectly regulate microbial communities. Two orders of mites are generally distinguished: the acariforms and the parasitiformes [24]. Some are predators (mainly parasitiforms) and others are parasites (of plants or animals) or decomposers (mainly acariforms). Some species carry bacteria and fungi on their surface, thus contributing to their dissemination.
3.3. Macrofauna
Another functional group, not related to a trophic group, is also often used; these are the “soil engineers” [26]. Soil engineers modify the soil environment through their construction activities (grubs, galleries, nests) that affect microbial diversity and activity. Soil engineers are mainly represented by earthworms (endogeous and anecitic) and ants. Earthworm species have been grouped into three categories:
- endogeous (small geophagous worms living permanently in the first centimeters of soil in a network of horizontal galleries).
- aneciques (a strange term for large saprophagous worms living in large galleries that can go down to a depth of 3 m, the “ploughmen” of the soil).
- epigeneans (small red worms living in the litter and generally not digging galleries) [27].
4. Measuring biodiversity
Over the past few decades, many research programs on soil biodiversity have sought to identify relevant indicators, to produce a comprehensive mapping and/or to identify soil uses influencing soil biodiversity.
Among the methods of researching functional characteristics of organisms, the one called “ecological traits and preferences” is very relevant to describe the functions of communities. Thus, for example, a fungal community can be characterized by its capacity to mineralize different molecules (e.g. sugars, amino acids, polymers), or by its membership in a trophic group (e.g. saprophytes, symbiotics, endophytes, pathogens; see section 3.2). Or, an earthworm community can be characterized by the lifestyle of the different species or by the length of the individuals. It is in this context that the TEBIS (Traits Écologiques et Biologiques des organismes du Sol) network was created, in order to better characterize the ecological traits and preferences of soil organisms, as bioindicators in particular.
This article has modestly attempted to show that the different biotic compartments of the soil interact with each other. Thus, by developing simultaneous studies of different biotic compartments of soils subjected to different uses and at different times, we will be able to have a more realistic view of the biodiversity of soils and the interactions between the different organisms in this ecosystem. Only this global approach studying the whole community (and not isolated biotic components) allows to consider all biological functions simultaneously [32], [33].
Messages to remember
- A quarter of the total biodiversity of the planet is found in soils.
- Soil biodiversity can be classified into 4 major groups: micro-organisms, micro-fauna, meso-fauna, macro-fauna. Plants can also be considered through their root system.
- The different soil organisms(taxonomic diversity) have essential roles (functional diversity) in soil functioning.
- The roles of these organisms in the soil are, for the most part, related to their biological interactions (prey-predator relationship, symbiosis etc.).
- Many factors simultaneously modify the state of soil biodiversity, such as use, seasons, biotic interactions, etc.
- Soil biodiversity must be considered in a global way, taking into account the close relationships and strong impacts between its different biological components.
Notes and references
Cover image. [Source : © A.Auclerc]
[1] Jeffery, S., Gardi, C., Jones, A., Montanarella, L., Marmo, L., Miko, L., Ritz, K., Peres, G., Römbke, J., Putten, W.H. van der, 2010. European Atlas of Soil Biodiversity, European Commission, Publications Office of the European Union. Publication Office of the European Union.
[2] Lavelle, P., Spain, A.V. 2001. Soil ecology. Vadose Zone Journal
[3] Sandlund et al. 1993. Quoted by RAMADE F., 2002, Dictionnaire encyclopédique de l’écologie et des sciences de l’environnement, Paris, Dunod, 1075 p.
[4] Vincent, Q., 2018. Study of abiotic, biotic and functional parameters and their interactions in neglected soils. PhD thesis. University of Lorraine.
[5] Bertrand, J.-C., Caumette, P., Lebaron, P., Matheron, R., Normand, P., 2011. Microbial ecology: Microbiology of natural and anthropized environments. Presses universitaires de Pau et des Pays de l’Adour
[6] Karimi, B., Chemidlin Prévost-Bouré, N., Dequiedt, S., Terrat, S., Ranjard, L., 2018. Atlas Français des Bactéries du Sol.
[7] Roesch, L.F.W., Fulthorpe, R.R., Riva, A., Casella, G., Hadwin, A.K.M., Kent, A.D., Daroub, S.H., Camargo, F.A.O., Farmerie, W.G., Triplett, E.W., 2007. Pyrosequencing enumerates and contrasts soil microbial diversity. ISME J. 1, 283-290.
[8] Janssen, P.H., 2006. Identifying the dominant soil bacterial taxa in libraries of 16S rRNA and 16S rRNA genes. Appl. Environ. Microbiol. 72, 1719-1728.
[9] Gobat, J.-M., Aragno, M., Matthey, W., 2010. Le sol vivant: bases de pédologie, biologie des sols, 3rd ed. PPUR Presses polytechniques.
[10] Ahemad, M., 2014. Remediation of metalliferous soils through the heavy metal resistant plant growth promoting bacteria: Paradigms and prospects. Arab. J. Chem. nd.
[11] Bardgett R.D . & D. Wardle. Aboveground-Belowground linkages. Biotic interactions, ecosystem processes, and global change. Austral Ecology 37: 26-27. 2010
[12] Clarholm M. Interactions of bacteria, protozoa and plants leading to mineralization of soil nitrogen, Soil Biol. and Biochem. 17 : 181-187. 1985.
[13] Tedersoo, L., Bahram, M., Polme, S., et al., 2014. Global diversity and geography of soil fungi. Science.
346, 1256688-1256688.
[14] Toju, H., Kishida, O., Katayama, N., Takagi, K., 2016. Networks depicting the fine-scale co-occurrences of fungi in soil horizons. PLoS One 11, 1-18.
[15] Schwarze, F., Engels, J., C., M., 2000. Fungal strategies of wood decay in trees, Springer, Berlin, Heidelberg.
[16] Meier, S., Borie, F., Bolan, N., Cornejo, P., Meier, S.A., 2012. Phytoremediation of metal-polluted soils by arbuscular mycorrhizal fungi. Environ. Sci. Technol. 7, 741-775.
[17] Narayanasamy, P., 2011. Microbial plant pathogens-detection and disease diagnosis, in: Microbial Plant Pathogens-Detection and Disease Diagnosis. pp. 1-256.
[18] Fortin, J.A., Plenchette, C., Piché, Y. 2016. Mycorrhizae: the rise of the new green revolution. Eds Quae
[19] Chadha, N., Mishra, M., Prasad, R., Varma, A., 2014. Root endophytic fungi: Research update. J. Biol. Life Sci. 5, 135.
[20] Nguyen, N.H., Song, Z., Bates, S.T., Branco, S., Tedersoo, L., Menke, J., Schilling, J.S., Kennedy, P.G., 2016. FUNGuild: An open annotation tool for parsing fungal community datasets by ecological guild. Fungal Ecol. 20, 241-248.
[21] Bachelier, G., 1971. Animal life in soils. http://www.vertcarbone.fr/wp-content/uploads/2018/03/Les-Animaux-du-sol-1.pdf
[22] Decaëns, T., Jiménez, J.J., Gioia, C., Measey, G.J., Lavelle, P., 2006. The values of soil animals for conservation biology. Eur. J. Soil Biol. 42, S23-S38.
[23] Orgiazzi, A., Bardgett, R.D., Barrios, E., Behan-Pelletier, V., Briones, M.J.I., Chotte, J.-L., De Deyn, G.B., Eggleton, P., Fierer, N., Fraser, T., Hedlund, K., Jeffery, S., Johnson, N.C., Jones, A., Kandeler, E., Kaneko, N., Lavelle, P., Lemanceau, P., M, D.H., 2016. Global soil biodiversity atlas, Global soil biodiversity atlas. European Commission.
[24] Dabert, M., Witalinski, W., Kazmierski, A., Olszanowski, Z., Dabert, J., 2010. Molecular phylogeny of acariform mites (Acari, Arachnida): Strong conflict between phylogenetic signal and long-branch attraction artifacts. Mol. Phylogenet. Evol. 56, 222-241.
[25] Hedde, M., van Oort, F., Renouf, E., Thénard, J., Lamy, I., 2013. Dynamics of soil fauna after plantation of perennial energy crops on polluted soils. Appl. Soil Ecol. 66, 29-39.
[26] Ruiz-Camacho, N., 2004. Biological Index of Soil Quality (BISQ): Bio-indicator of soil quality based on the study of macroinvertebrate populations.
[27] Bouche, M.B., 1972. Lombricians of France, Annals zool. Ecol. Anim. Annals zool. Ecol. Anim.
[28] Lavelle P., C. Lattaud, D. Trigo, I. Barois. Mutualism and biodiversity in soils. In: The significance and regulation of soil biodiversity. p23-33. Springer, Dordrecht. 1995
[29] Cluzeau D., M. Guernion, R. Chaussod, F. Martin-Laurent, C. Villenave, et al. Integration of biodiversity in soil quality monitoring: Baselines for microbial and soil fauna parameters for different land-use types. European J. Soil Biol. 2012:.63-72. hal-00704897.
[30] Vincent Q, C Chartin, I Krüger, B van Wesemael, M Carnol. Guide on biological indicators and organic carbon of agricultural soils in Wallonia-Biological quality and organic carbon of agricultural soils in Wallonia. Ed. DGARNE. SPW (B).
[31] Ponge J.F. 2012. https://www.futura-sciences.com/planete/dossiers/developpement-durable-sol-biodiversite-etonnante-966/
[32] S Soliveres, S., van der Plas, F., Manning, P., et al., 2016. Biodiversity at multiple trophic levels is needed for ecosystem multifunctionality. Nature. 536, 456-459
[33] Creamer, R.E., Hannula, S.E., Leeuwen, J.P.V., et al., 2016. Ecological network analysis reveals the inter-connection between soil biodiversity and ecosystem function as affected by land use across Europe. Appl. Soil Ecol. 97, 112-124.
The Encyclopedia of the Environment by the Association des Encyclopédies de l'Environnement et de l'Énergie (www.a3e.fr), contractually linked to the University of Grenoble Alpes and Grenoble INP, and sponsored by the French Academy of Sciences.
To cite this article: VINCENT Quentin, AUCLERC Apolline, LEYVAL Corinne (May 16, 2022), Soil biodiversity, Encyclopedia of the Environment, Accessed April 26, 2024 [online ISSN 2555-0950] url : https://www.encyclopedie-environnement.org/en/soil/soil-biodiversity/.
The articles in the Encyclopedia of the Environment are made available under the terms of the Creative Commons BY-NC-SA license, which authorizes reproduction subject to: citing the source, not making commercial use of them, sharing identical initial conditions, reproducing at each reuse or distribution the mention of this Creative Commons BY-NC-SA license.