Solar energies
PDF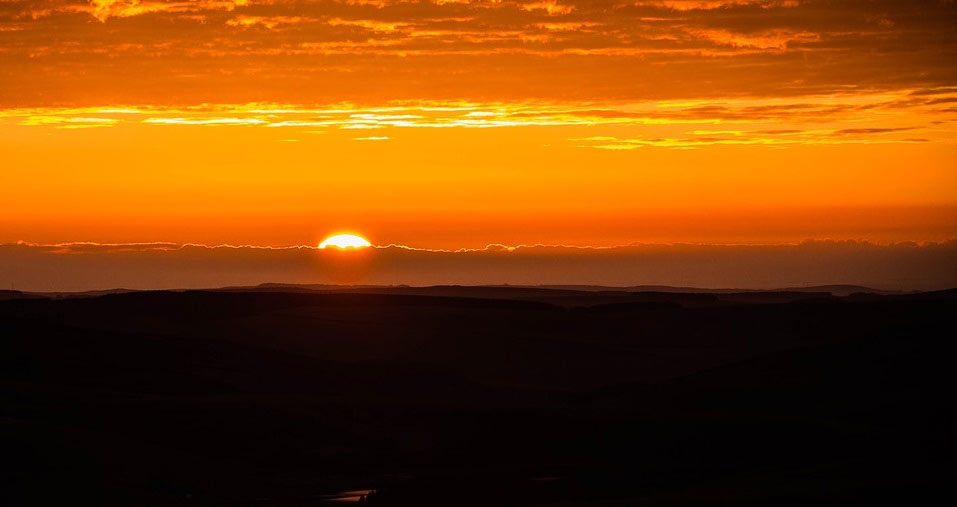
Sun radiation is commonly referred to as solar energy, limiting this energy to visible radiation. But the Sun sends us energy in many forms: radiation in all wavelengths, but also ions and electrons in the form of a “solar wind”. What are these various sources of solar energy perceived on Earth? What is their origin?
To think “solar energy” is, most often, to think of its light, i.e. photons, particles of light associated with all electromagnetic radiation, from gamma rays to visible rays according to wavelength (read The thermal radiation of the black body and The Colours of the Sky). They are emitted during changes in the energy levels of atoms and ions where there is a relatively highly excited material, especially in stars, and circulate in the interplanetary void. Often, this flux is implicitly restricted to its visible component, with a wavelength between 0.4 (purple) and 0.7 (red) micron (μm). This vision is too restrictive. It would be more appropriate to talk about solar energy…
1. A boiling source of photons
This measurement is one of the very first results of a branch of astrophysics called solar seismology, a discipline that has proved to be one of the most fruitful in recent years by focusing on explaining all the “eigenmodes” (this 5-minute period constitutes one of the Sun’s “eigenmodes”).
2. A variety of radiations
What radiation escapes from the photosphere? It is a radiation that took millions of years to come out of the star, that has been absorbed, re-emitted countless times and, as a result of collisions, has considerably broadened its spectrum (see The Colours of the Sky). Radiation can be characterized in several ways: by its wavelength, or by its energy. The energy is inversely proportional to the wavelength. The higher the wavelength, the lower the radiation energy. In the article Space weather and its consequences on Earth we give the correspondence between energies and wavelengths.
Most of the solar emissions are found in the visible range. The most intense colour is blue-green, which corresponds to a central wavelength of this visible band, close to 0.5 micron (μm). But as all colours coexist, their mixture results in the very bright yellow to which we are accustomed and which hardly changes over time: the energy contained in this visible part of the spectrum probably varies by less than 0.1% over a decade.
However, this variation is not insignificant: it could be the cause of regional climatic phenomena on Earth, such as the small Ice Age from 1550 to 1750, which brought winters so cold that the main French rivers froze sufficiently to be used as transport routes with carriages pulled by teams. Paris keeps a record of it, by the name of the rue de la Glacière in the 13th arrondissement [1] (read Variability of solar activity and climate impacts).

The Sun also emits radiation outside the visible range. For example, waves in microwave frequencies (long wave radio waves), the same ones we use for radio and television. These do not interfere with everyday life in any way, and have the same effect on human skin as an electric lamp because these waves are of low energy. Another example: when exposed to the sun, the skin tans (or burns). Everyone has heard that this is an effect of ultraviolet rays, from which we must protect ourselves (see Cellular Impact of Solar UV). These waves have a short wavelength, and therefore a high energy per photon. Most of this radiation is emitted in a layer outside the photosphere, the solar corona.
Ultraviolet is only part of the energy radiation. The Sun emits even more energetic waves: extreme ultraviolet, X-rays, gamma rays. In both the radio and ultraviolet bands, the amount of photons emitted is small compared to the emission in the visible, and the energy collected there represents just over 1 ten millionth (or 0.0000001%) of the total energy. Figure 2 shows how the energy of solar radiation is distributed according to wavelength.
The main characteristic of this radiation outside the visible part is that it varies over time, with a remarkable periodicity. First of all, the sun emits little, and then, within about five and a half years, the intensity of this radiation increases tenfold, then decreases and returns to its original state. This flood and recession define a “solar cycle”, or Schwabe cycle. There are other solar cycles, ranging from a few hours to several hundred years.
The total power radiated by the Sun, all rays combined, is about 4 x1026 Watt (about 260 million Watts per square meter (W/m2) of solar surface; 1 W = 1 J/s = 1 N.m/s). Above the clouds, the Earth receives only a tiny fraction: 1.743 x 1017 Watts, or 1361 W/m2 perpendicular to the Sun (see The Atmosphere and the Earth’s Gas Envelope). This last number has been called the solar constant in the past, despite the fact that it is not as constant as thought before. Today we speak of total solar irradiance.
3. The source of the solar wind
From the photosphere, our eyes perceive visible radiation, which is therefore only a part of all the radiation emitted. But even more: the photosphere is the place where solar matter is expelled, a kind of permanent evaporation that creates what has been nicely described as the “solar wind“. What is it about?
The outermost layer of the solar interior, the “convective zone”, is constantly boiling. Too far from the centre to be compacted by gravity, not dense enough to trigger nuclear reactions, it has a strong dynamic. However, the matter of which it is composed is ionized, i.e. it consists not of atoms, but of ions (charged particles) which often follow a relatively coherent movement, and of electrons, mobile, light, which go everywhere while ensuring the electrical neutrality of the whole. This is called a “plasma“, the fourth state of nature next to the gaseous, liquid and solid states. A plasma is distinguished from common matter by the fact that it is sensitive to the presence of an electromagnetic field and that its movement itself generates an electromagnetic field (by dynamo effect). The base of the convective zone is called the tachocline. The tachocline is a boundary where the moving plasma of the convective zone rubs against a more internal zone of the Sun, in which the plasma is compact and free of internal movements: the radiative zone. The tachocline is the source of the solar magnetic field.
This description is that of an observer rotating coherently with the Sun. For an observer outside the star, these particle movements are complemented by the Sun’s own rotation, which averages 27 Earth days. The effect is quite the same as that of the movement printed with water expelled from a rotating garden watering can: an ant on the watering can sees the water expel itself straight (radially), but the gardener sees it describe a spiral. Seen from the Earth, the interplanetary magnetic field does not seem to come straight from the Sun, but from its edge, from where it whips the space environment into a graceful curve. The average characteristics of the solar wind at Earth level are a speed of 370 km/s and a concentration of 5 million particles per cubic meter, i.e. an extreme vacuum!
From 1995 to 2009, a satellite, Ulysses, manufactured by the European Space Agency (ESA), revolved around the sun from pole to pole. His measurements, taken at a distance slightly greater than the Sun-Earth distance, revealed two solar wind regimes. Between 20° south and 20° north of heliospheric latitude, the solar wind flows with an average velocity of 370 km/s, which is subject to wide variations. Then, in less than ten degrees, the speed increases to 750 km/s. The regime then becomes fast, but much less disrupted. Then the speed increases slowly to reach about 80° of latitude at about 800 km/s. But five and a half years later, we can see that all around the Sun, the two solar wind regimes, the “slow” and the “fast” are strongly intertwined. In the first case, the sun is called “calm”. In the second, it is referred to as “active”. It takes ten to thirteen years to return to an initial situation. This period is called a “solar cycle”, or “Schwabe cycle” already mentioned.
As we can see, solar activity is defined in various ways, some of which are not described in this article (solar flares, coronal mass ejections, coronal holes, sunspots…) entirely devoted to the solar energies received on Earth. All manifestations of solar activity have a common origin: variations in the star’s magnetic field, which have only begun to be well understood since the beginning of the twenty-first century.
On average, the solar wind causes the Sun to lose 1014 kg per day, only four times less than the mass lost as radiation in the nuclear reactions of the core. Since the solar mass is 2 x 1030 kg, it would take more than five hundred billion centuries to completely empty our star through this process, which is much higher than the solar life expectancy of 5 billion years. In the end, the mass ejected in the form of a solar wind is totally negligible.
References and notes
[1] J. Lilensten, T. Dudok de Wit, K. Matthes, Earth’s climate response to a changing Sun, ISBN 978-2-7598-1849-5, DOI: 10.1051/978-2-7598-1733-7, EDPS Ed., 2015
The Encyclopedia of the Environment by the Association des Encyclopédies de l'Environnement et de l'Énergie (www.a3e.fr), contractually linked to the University of Grenoble Alpes and Grenoble INP, and sponsored by the French Academy of Sciences.
To cite this article: LILENSTEN Jean (March 7, 2024), Solar energies, Encyclopedia of the Environment, Accessed July 27, 2024 [online ISSN 2555-0950] url : https://www.encyclopedie-environnement.org/en/air-en/solar-energies/.
The articles in the Encyclopedia of the Environment are made available under the terms of the Creative Commons BY-NC-SA license, which authorizes reproduction subject to: citing the source, not making commercial use of them, sharing identical initial conditions, reproducing at each reuse or distribution the mention of this Creative Commons BY-NC-SA license.