The fixed life of plants and its constraints
PDF
Plants are fixed to the soil by their roots, which supply them with water and minerals, their leaves capturing solar energy to fix the carbon from carbon dioxide. These essential processes of earthly life are therefore carried out by immobile organisms. Plants must therefore be able to adapt to the contrasting and fluctuating conditions of their environment, without the possibility of finding a more favourable habitat than movement would allow them, as is the case with animals. Evolutionary forces have helped to shape the development and physiology of plants to adapt to the different climatic zones of the planet, from taiga to desert, through tropical or temperate zones. The resulting plant biodiversity is of enormous richness. But environmental variations in the same place on our planet can also fluctuate greatly with the seasons. Plants of the same species then acquired cellular and molecular mechanisms allowing them to perceive external changes and reprogram the expression of their genome. They can thus redirect their development, physiology and metabolism in order to adapt as effectively as possible to these changes
1. Plants: sessile organisms that adapt without moving
The word adaptation comes from the Latin adaptare. It can be defined as a set of adjustments or changes in the behaviour, physiology or structure of an organism that enable it to become better able to live in a defined environment.
Whether it is windy, rainy, snowy, freezing to death or that a scorching heat wave overwhelms us… the plants are there! It is indeed one of their characteristics to adapt to highly fluctuating environmental conditions. Plants have to deal with very large differences in temperature, light and humidity depending on the time of day, the seasons and the places where they grow. The nature of the soil also determines particular conditions for plant growth and development, and significant deficiencies in mineral nutrients (nitrogen, phosphorus, etc.) may exist in the soil, or, in contrast, harmful toxicities due to excess toxic metals (cadmium, lead, aluminium, etc.) may occur. Some irrigation water, or land by the sea, causes saline stressStress caused by soil salinity. This salinity can be natural or induced by agricultural activities such as irrigation (with low quality water) or the use of certain types of fertilizers. disrupting normal plant nutrition processes. These fluctuations in the physical environment favour the geographical distribution of plants according to their ability to adapt to a biotopeLocation with relatively uniform determined physical and chemical characteristics. This environment is home to a set of life forms that make up biocenosis: flora, fauna, micro-organisms. A biotope and the biocenosis it supports form a given ecosystem. There are shade plants such as ferns, which prefer to grow out of the light, or aquatic plants such as the élodée, which require a lot of water. Similarly, calcareous soil will support calcareous plants “that settle in limestone”. This is the case for garrigue plants in the south of France. Plants that “flee from limestone” or calcifuges, such as chestnut trees or ferns, prefer acidic soils. But plants do not only interact with their physical environment. They also interact with other living organisms. Some may be useful to them by promoting their nutrition, for example, symbiotic bacteria and fungi {tooltip}mycorrhizal fungi{ind-text}Mycorrhizal relatives, which are symbiotic associations between the roots of plants and soil fungi. Mycorrhizae affect more than 95% of terrestrial plants. They give plants better access to soil nutrients and help them better resist environmental stresses.. Others are harmful to them by infecting them, such as viruses, bacteria, phytopathogenic fungi, or by eating them, as is the case with many insects and herbivores in general. Just as plants have adapted to the physical variations of their environment, they have, over the course of evolution, developed responses to defend themselves against the aggression of pathogens.
Unlike animals, plants do not flee to avoid adverse or aggressive conditions that jeopardize their integrity or survival. They do not have the central nervous system that allows animals to analyze the information their senses provide them, triggering actions to adapt to changing situations. They are fixed to the soil by their roots, which provide the aerial parts with water and essential mineral elements: nitrogen, phosphorus, potassium, sulphur, iron, zinc, magnesium, manganese… [3]. Leaves are able to transform the light energy provided by the sun into carbonaceous organic molecules (sugars, lipids, proteins) through the reaction of photosynthesisBioenergetic process that allows plants, algae and certain bacteria to synthesize organic matter from the CO2 in the atmosphere using sunlight. Solar energy is used to oxidize water and reduce carbon dioxide in order to synthesize organic substances (carbohydrates). The oxidation of water leads to the formation of O2 oxygen found in the atmosphere. Photosynthesis is at the base of autotrophy, it is the result of the integrated functioning of the chloroplast within the cell. [4] (link to article Light on photosynthesis). Briefly, let us recall that photosynthesis occurs in leaf specific cellular organellesSpecialized structures with a specific function within the cell. For example, the nucleus, mitochondria and chloroplasts, the chloroplastsOrganites of the cytoplasm of photosynthetic eukaryotic cells (plants, algae). As a site of photosynthesis, chloroplasts produce O2 oxygen and play an essential role in the carbon cycle: they use light energy to fix CO2 and synthesize organic matter. They are thus responsible for the autotrophy of plants. Chloroplasts are the result of the endosymbiosis of a photosynthetic prokaryote (cyanobacterium type) within a eukaryotic cell, about 1.5 billion years ago.. Their chlorophyll captures solar photons leading to the cleavage of water molecules and the release of oxygen, the assimilation of carbon from carbon dioxide (CO2) into organic molecules, and the production of chemical energy (Adenosine Triphosphate or ATP Abbreviation of adenosine triphosphate. A triphosphate nucleoside composed of adenine (nitrogen base), ribose (sugar with 5 carbon atoms) and three phosphate groups forming a triphosphate group. A compound that both donates and stores energy present in all living organisms. Also used as building materials for nucleic acid synthesis.).
Plants, because of their fixed life and their lack of sensory organs for perceiving the outside world on the one hand, and of a central nervous system on the other, have therefore evolved to adapt to contrasting environmental conditions in space and fluctuating over time.
These two aspects of plant adaptation do not use the same concepts and mechanisms. In the first case, it is the spatial adaptation of different plant species to the different climates of the planet. Not all plant species grow everywhere, and this climato-geographical adaptation has been based on the principles of natural selection of beneficial traits, traits that have become established over time in the genetic heritage of the species (see The adaptation of organisms to their environment). The second case involves the adaptation of plants of the same species to fluctuating environmental conditions in a given place. A plant can thus successively undergo periods of drought, cold, high light intensity, etc. It adapts to these variations by activating physiological processes, often using reprogramming of gene expression leading to high phenotypic plasticityThe ability of an organism to express different phenotypes from a given genotype under environmental conditions. (see Adaptation: responding to environmental challenges).
It is therefore the combination of characteristics fixed in the genome of the different plant species and the plasticity of their genome expression that allows plants to cope, without moving, with very diverse environmental conditions.
2. Spatial adaptation of plants: from desert to taiga
Plants can be found all over the world, at all latitudes and longitudes, and at all altitudes. However, the conditions of temperature, luminosity and hygrometry are extremely variable in different climates. As dry as deserts are, tropical forests are full of water! It is enough to observe the plants that grow in these extreme environments to realize that they often do not belong to the same species and that they have morphological and anatomical characteristics very characteristic of the environment in which they grow [8].
Let us take two examples of radically different landscapes: the taigaForest-type plant formation traversed by a vast lake system resulting from fluvioglacial erosion. Strongly linked to the subarctic climate, it is one of the main terrestrial biomes. It is a transition zone between the boreal forest and the Arctic tundra, Siberian and the Arizona desert (Figure 4).
A desert is a very dry region of the globe, characterized by rainfall of less than 200 and often even 100 mm/year, marked by poor soils and scarce plant populations. This lack of water is associated with irregular rainfall from one year to the next. Deserts are found at all latitudes and longitudes and cover about a third of the land surface, almost 100 times the size of France. They spread mainly across the Tropic of Cancer, in Western Asia, in the interior of Australia, and at polar latitudes. The common feature of all deserts is the lack of water. The low relative humidity of the air (generally less than 50%) and the clear sky most often explain the high temperature variations. In hot deserts, temperatures above 50°C during the day are followed by temperatures below 0°C at night.
In deserts, no coniferous trees! The flora is very particular (Figure 4), and perfectly adapted to arid conditions where water is the most precious commodity. This is the territory of succulent plantsQuality of fleshy plants (fat plants, for example) adapted to survive in arid environments due to the characteristics of the soil and climate. such as cactus, which have reduced their leaf area to a minimum, sometimes to simple thorns, with stems providing photosynthesis activity (Figure 5). Plants living in deserts do so through three main modes of adaptation: succulence, tolerance to drought or drought avoidance. The so-called succulent plants, which include all species of the cactus family, have the particularity of being able to store water in their young leaves, stems or roots. They must be able to absorb very large quantities of water in a short time, as rains are often of low intensity and do not last long, as soils dry quickly under the influence of intense sunlight. Almost all succulents have very long roots, developing horizontally on the surface to capture water resources most efficiently. Once this water has been absorbed and stored, it should not be lost, which is made possible by the reduction in size or even the absence of leaves. In addition, these leaves and stems are covered with a waxy cuticle that makes them practically impervious to the outside environment. At the physiological level, many succulent plants have a very efficient photosynthesis mode with respect to water called CAM for “Crassulacean Acid Metabolism” (see Focus The House-leek). CAM plants open their stomata at night to facilitate gas exchange, and thus store carbon dioxide which will be used by photosynthesis during the day while the stomata are closed, thus limiting water losses. Due to lower temperatures and higher humidity at night, CAM plants lose 10% less water per unit of carbohydrates, compared to plants whose gas exchanges occur with stomata that are open during the day (so-called C3 plants).
Drought tolerance is an ability of many plants in drylands. These plants are capable of undergoing desiccationAction of drying out; drying out: drying out the soil, of a plant. Removal of water, natural or not, contained in a substance without dying. Often, they lose their leaves during dry periods and enter a deep dormancy. The greatest water loss of a plant is through evapotranspiration through the surface of the leaves and stomata; therefore, the loss of leaves helps to preserve water in the stems. Some plants do not have this ability to lose their leaves, which are covered with resins that limit water evaporation. Unlike succulent plants that have a superficial root system, some desert trees and shrubs survive thanks to a highly developed root system, which can reach twice the surface of the canopyUpper forest floor, directly influenced by solar radiation. Considered as a habitat or ecosystem as such, particularly in tropical forests where it is particularly rich in biodiversity and biological productivity. and this to great depths. When heavy rains occur, the deep soil remains wet longer, allowing these plant species to grow in longer time steps. On the other hand, this type of plant can maintain photosynthetic activity even if there is very little or no water, which would be fatal to most plants in temperate zones.
A third type of plant found in deserts… simply does not exist most of the time because the conditions are too unfavourable. This drought avoidance is possible in these annual plants, which use all their energy to produce seeds quickly, instead of maintaining their vegetative state for as long as possible. Fall conditions are often favourable in many deserts because of rainfall and falling temperatures. Non-dormantProperty of an organism with a slower life phase where growth and development are temporarily stopped. seeds from annual plants can germinate quickly and massively and complete their entire life cycle in a few weeks. They then produce enough seeds to ensure the sustainability of the species before winter conditions set in.
These two examples of specialized flora adapted to survival in contrasting and hostile environments (taiga and deserts) clearly illustrate the role of natural selection in the evolution of plant species best adapted to the particular, sometimes extreme, conditions of a given environment. Over time, the mutations that have allowed the development most adapted to external conditions (e.g. leaf morphology, Figure 5) have been fixed in the species’ genomes to ensure their sustainability in specific habitats.
3. The temporal adaptation of plants: storm warning!
Extreme cases of drought adaptation are also remarkable, such as Jericho roses (Selaginella lepidophylla), more commonly referred to as resurrection plants that appear to be dead and “live” very quickly if they receive water (Figure 7).
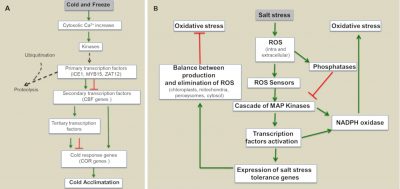
Two examples of signalling pathways used by plants to adapt to particular stress conditions, cold or excess salt, are presented in Figure 8. When the cold occurs, a cascade of events occurs in the plant and will regulate the expression of cold response genes. First, the calcium concentration of their cytosol increases, which leads to the activation of a number of enzymes that modify transcription factors [10]. These factors then attach themselves to the DNA upstream of cold response genes, or their regulators, to activate or suppress their expression. When stress stops, the system returns to equilibrium through other post-transcriptional changes in certain transcription factors that cause them to be degraded by proteolysis (Figure 8A).
The response of plants to excess salt in their environment is determined by a balance between the production and elimination of activated forms of oxygen (ROS) such as hydrogen peroxide (H2O2), superoxide ion (O2.-) or the hydroxyl radical (-OH). The perception of these ROS by sensors activates kinases that will phosphorylate transcription factors, and thus activate them. The products of the response genes regulated by these transcription factors will lead to the elimination of ROS and therefore oxidative stress caused by excess salt. The balance is also determined by the fact that the activation of phosphatases will counterbalance the action of kinases, and that, conversely, the activation of oxidases will promote oxidative stress.
Many variations of the schemes presented in Figure 8 exist to reflect the specificity of a given stress, but the following general principles that are now widely accepted in the scientific community can be stated:
- Stress will generate the production of signals by the plant.
- These signals are often small organic molecules from metabolic activity, they can be:
– polysaccharides derived from the degradation of plant walls;
– lipid molecules resulting from the action of specific enzymes such as lipoxygenases, enzymes that catalyse the oxidation of fatty acids;
– small peptides capable of circulating in the sap developed to signal stress at long distances in the plant.
Some of these metabolites act as plant hormones, such as abscisic acid, which is considered a true stress hormone. - These signals are perceived by receptors that are often proteins located in the cell membrane; they have kinase or phosphatase activities, i.e. they remove or add phosphate groups.
- In many cases, the propagation or amplification of signals requires the intervention of secondary messengers. Ca2+ ions and activated oxygen species (ROS) are the secondary messengers most regularly involved in plant responses to environmental variations.
- These secondary messengers enable the activation of cascades of protein kinases and phosphatase proteins soluble in the cytoplasm and nucleus of cells.
- in the end, the terminal targets of these reaction cascades are often transcription factors, capable of binding to DNA upstream of stress response genes whose expression they activate.
- All the products of these genes (structural proteins, enzymes, etc.) allow the adaptive phenotypic response of plants to the stress they undergo.
In addition to this regulation of gene expression in response to environmental constraints, there is also epigenetic regulation [11],[12] (read Epigenetics, the genome and its environment & Epigenetics: How the environment influences our genes). As environmental variations often occur repeatedly, it is advantageous for plants to have a “memory” of these past events, and to use the storage of this information to adapt more effectively to new episodes. One of the best known examples concerns defence against herbivores, but these mechanisms also concern adaptation to abiotic stresses. Different means allow this memorization: accumulation of metabolic compounds, such as osmoprotectants to resist drought, phosphorylation / dephosphorylation of regulatory proteins as mentioned above. But much research has highlighted the importance of epigenetic regulation in adapting to different stresses, and in particular the role that small regulatory RNAs called miRNA and siRNA can play. Initially, epigenetic regulations allowing plants to adapt to environmental constraints were described in the case of adaptation to poor phosphorus and copper nutrition conditions. The role of these small RNAs has since been clarified for adaptation to drought or temperature increases. At a more integrated level, genes carried by DNA are packaged in the cell nucleus in a complex combining DNA and proteins called chromatin. The state of compaction of the chromatin determines the expression of genes. It is regulated by post-transcriptional modifications (methylation, acetylation, phosphorylation, etc.) of histones, proteins that structure DNA within chromatin (see Epigenetics, the genome and its environment). The stress conditions for the plants mentioned above are thus able to modify the structure of the chromatin in the vicinity of genes important for adaptation to these stresses. This process therefore contributes to the regulation of the expression of their stress genes and the adaptive response of plants [13].
4. The future of plant adaptation in the context of climate change
The climate change that our planet is currently experiencing is manifested by, among other things, temperature increases, a change in precipitation patterns and an increase in the concentration of CO2 in the atmosphere. Drought and floods are known to influence plant biology. The adaptive phenomena of plants will therefore necessarily evolve in a multi-stress context with the increase in atmospheric CO2 as a determining element [14].
Several studies have analysed the transcriptome (the mRNA repertoire, i.e. expressed genes), proteome (the protein repertoire) and metabolome (the metabolite repertoire) of different species exposed to high concentrations of CO2. Significant reprogramming at all these levels has been observed and mainly concerns photosynthesis and carbonaceous metabolism, as well as the biosynthesis of amino acids, starch and sugars. Another parameter that is being profoundly altered by climate change is plant nutrition. The increase in temperature and CO2 concentration will affect the physiology of soil microorganisms and thus alter nutrient cycles and their availability for plant growth [15]. Experiments were thus carried out on plants grown at CO2 concentrations equivalent to those expected in 2050. They showed that, under these conditions, the iron and zinc concentrations of C3 plant seeds are significantly reduced. The protein concentration of C3 plants is also decreasing due to the alteration of nitrogen nutrition at high CO2 concentrations. However, plants with CAM metabolism are less constrained by these CO2 increases.
This climatic evolution will therefore have the consequence of modifying the geographical distribution of certain species, and promoting the emergence of new adaptive processes, but also of influencing human activity by modifying the nutritional quality of plants [16]. This will have an impact on agricultural practices.
References and notes
Cover image. [Source: Assignment http://www.ForestWander.com License; License CC-BY-SA 3.0]
[1] http://bip88.net/bip/index.php/activites-humaines/agriculture/40Semaine-9 & http://bip88.net/bip/index.php/activites-humaines/agriculture/40Semaine-2
[2] https://www.youtube.com/watch?v=9wLnavgmVjs
[3] https://fr.wikipedia.org/wiki/Nutrition_v%C3%A9g%C3%A9tale
[4] https://fr.wikipedia.org/wiki/Photosynth%C3%A8se
[5] http://acces.enslyon.fr/evolution/evolution/relations-de-parente/enseigner/activites-pratiques-et-classification/les-tp/tp-presence-de-stomates
[6] http://www.cours-pharmacie.com/biologie-vegetale/leau-de-labsorption-a-la-transpiration.html
[7] http://www.snv.jussieu.fr/bmedia/mouvements/nasties-stomate.htm
[8] http://www.mbgnet.net/bioplants/adapt.html
[9] http://www.bdesciences.com/nolaj/SVS/L3/Semestre%20VI/Replies%20of%20Plants%20a%20l%20Environment/Courses/Krys3000%20%282010%29/II%20-%20R%C3%A9response%20of%20plants%20to%20stress%20abiotics.pdf
[10] Transcription factor: protein necessary for initiating or regulating the transcription of DNA into RNA molecules.
[11] Tetsu Kinoshita T & Seki M (2014) Epigenetic Memory for Stress Response and Adaptation in Plants. Plant & Cell Physiology 55: 1859-1863. doi:10.1093/pcp/pcu125
[12] Sunkar R, Chinnusamy V, Zhu J & Zhu JK (2007) Small RNAs as bigplayers in plantabiotic stress responses and nutrient deprivation. Trends in Plant Science 12:301-309
[13] Kim JM, Sasaki T, Ueda M, Sako K & Seki M (2015) Chromatin changes in response to drought, salinity, heat, and cold stresses in plants. Frontiers in Plant Science 6:114. doi: 10.3389/fpls.2015.00114
[14] Ishita Ahuja, Ric CH de Vos, Atle M. Bonesand Robert & D. Hall (2010) Plant molecular stress responses face climate change. Trends in Plant Science 1:664-674. doi:10.1016/j.tplants.2010.08.002
[15] Pilbeam DJ (2015) Breeding crops for improved mineral nutrition under climate change conditions. Journal of Experimental Botany, 66:3511-3521. doi:10.1093/jxb/eru539
[16] Myers SS et al. (2014) Increasing CO2 threatens human nutrition. Nature 510:139-142. doi:10.1038/nature13179
The Encyclopedia of the Environment by the Association des Encyclopédies de l'Environnement et de l'Énergie (www.a3e.fr), contractually linked to the University of Grenoble Alpes and Grenoble INP, and sponsored by the French Academy of Sciences.
To cite this article: BRIAT Jean-François (December 5, 2019), The fixed life of plants and its constraints, Encyclopedia of the Environment, Accessed July 27, 2024 [online ISSN 2555-0950] url : https://www.encyclopedie-environnement.org/en/life/fixed-life-of-plants-and-its-constraints/.
The articles in the Encyclopedia of the Environment are made available under the terms of the Creative Commons BY-NC-SA license, which authorizes reproduction subject to: citing the source, not making commercial use of them, sharing identical initial conditions, reproducing at each reuse or distribution the mention of this Creative Commons BY-NC-SA license.