逆温层、雾和低层大气的其他奇特现象

为什么每年二月到三月,反气旋存在的情况下,山谷中海拔较高的区域反而比被云海覆盖的谷底温度更高?这种情况与对流层中大气温度随高度上升线性下降的规律恰恰相反,其原因在于逆温层的存在。什么是逆温层?这种现象产生的机理是怎样的?它受哪些参数控制?又会产生什么样的结果?如我们所见,逆温层一般位于地表或高空,除了导致雾的形成,这一现象还具有某些不为常人所知的影响。例如,在接近地面的地方,逆温层的存在会扩大声波或电磁波的传播范围。
1. 什么是逆温层?
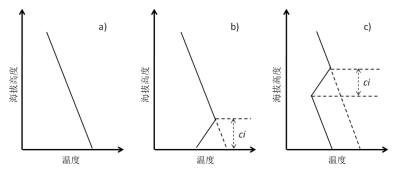
在存在高压系统的情况下,对流层中的大气通常非常接近标准大气:气压和密度随高度呈指数下降,温度随之以6.5℃/km的速率线性下降(详见《地球的大气层和气体层》)。气温之所以会随着海拔高度的升高而逐渐降低[1],是因为地球表面受到太阳辐射加热后,会通过传导和对流的形式,将热量传递给周围的空气。
然而,例外时有发生,当温度不以6.5℃/km的速率线性下降时,就会形成逆温层,导致局部地区温度随海拔的增加而升高(图1)。逆温层的厚度变化很大,从几十米到数百米不等,但它们的水平范围很广,也因此不负“层”这一定义。此外,逆温层的高度也是可变的,既可以发生在与地面直接接触的低层,也可以发生在地面与山峰之间的中层,更可以向上触及山顶。逆温层的厚度和高度很大程度上取决于其形成的机制。
在逆温层中,底部的空气比顶部的空气冷,故密度也更高。因此,这些逆温层非常稳定,不会发生对流运动和湍流。正是因为这种稳定性,逆温层通常被视为一种封层,防止下方堆积的物质或颗粒物(如花粉、化学污染物和空气污染颗粒物等)大量向上传输。
2. 土壤表面的逆温层

[来源:https://www.meteocontact.fr/pour-aller-plus-loin/les-inversions-thermiques]
每晚没有阳光的时候,地面或水体都会向外界发出红外线辐射,从而使自身冷却下来。与地表接触的空气相比于其上方的空气更冷、更重,因此没有上升的动力。因此,低层大气将保持静止,直到太阳升起。这种现象显而易见,因为太阳一下山,树叶和旗帜就停止了摇动,太阳一升起,它们又重新活跃起来。由于白天以对流为主的热交换已不复存在,这种夜间降温仅限于地面以上约100米的高度。这就在地表形成了一个逆温层,气温从地表向上逐渐升高,直至达到标准大气的正常值(图1b)。次日凌晨,太阳辐射导致地面和低层大气的温度再次升高。从而可能出现如图2所示的情况,非常冷的逆温层不再接触地面,而是高出地面几十米。此外,斜坡的方向也是影响因素之一,朝向东南的斜坡升温速度要快于谷底。
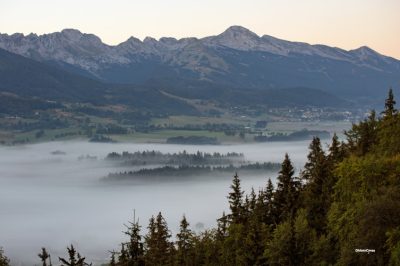
在北半球最冷的11月到3月,在这种静止的空气中,露点(dew point)[2]可以接近地面。空气中的水蒸气在夜间凝结成极小的液滴,并与空气混合,形成稳定且不会沉降的液态气溶胶系统——雾[3]。日出时,随着地面温度升高,低层空气变得更轻,进而抬升这些气溶胶,并压缩上层空气。该压缩过程既会加热上层空气[4],放大逆温效应,又会将地表上的雾压缩抬升,将其转变成层云(stratus cloud)(图3)。随着新一天的开始,雾层吸收太阳辐射,开始逐渐升温。当小液滴完全蒸发,雾就会完全消失。然而,在冬季,北欧一些纬度带的情况往往不是这样,只要反气旋条件持续存在,雾和逆温层就不会消失,而通常会持续长达几天。
3. 雾
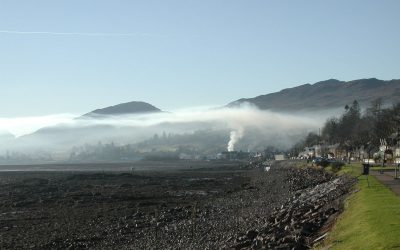
[来源:https://www.meteocontact.fr/pour-aller-plus-loin/les-inversions-thermiques]
雾中的液滴实际上是大量水分子的集合(assemblage),它们通常包裹着尺寸小于一微米的固体颗粒。这种同时含有固体和液体部分的物体通常被称为水汽凝结体(其形成条件详见《云层中发生了什么?》)。同悬浮在干燥空气中的微粒(详见《空气污染颗粒究竟是什么?》),特别是PM10和PM2.5一样,许多水汽凝结体的体积十分微小,因此可以悬浮在空气中。然而,值得注意的是,平均半径为几微米的水汽凝结体中往往能包含大约十亿个相距一纳米的水分子
为什么这种密度比周围空气大1000倍的颗粒能够在空气中保持平衡?具体原因如下:水汽凝结体受到两种相反的力:一是其自身重力,这是水汽凝结体下落的根本原因,二是初始位于其下方的空气摩擦力,这种力能够将水汽凝结体托举起来,与其重力作用相互抵消。重力与颗粒体积,即平均半径的立方(r3)成正比;摩擦力则与颗粒的外表面积,即平均半径的平方(r2)成正比。因此,当半径非常小时,重力相对于摩擦力就可以忽略不计,水汽凝结体就不能下落。另一方面,雨滴、雪花和冰雹之所以能下落至地面,是因为其尺寸大于重力和摩擦力之间的半径平衡阈值(约20 μm),平衡被打破,重力占据优势,迫使它们掉落下来。最重的颗粒下落的速度比那些半径接近平衡点的颗粒要快,因而形成小雨。
需要补充的是,当雾层形成时,其上层部分由于向外进行红外辐射而发生强烈的冷却。就像地面辐射一样,这有助于整个雾层的冷却。上述各种现象相结合,不仅会加强逆温层的逆温现象,也会导致逆温层的增厚。
各种污染物,无论是来源于工业的污染物,如富含碳氧化物和煤烟的烟雾、氮氧化物、甲烷、臭氧,还是来源于自然的污染物,如花粉(详见《空气污染》),都聚集在逆温层的下方或逆温层中较低的部分(图4)。同雾中的水汽凝结体一样,这些污染物因为没有对流运动或湍流而滞留在原地,并根据自身的不同性质,让雾呈现出灰色、蓝色或黄色。根据米氏散射定律(Mie’s Law of Scattering)[5](详见《天空的颜色》),产生这种颜色的原因是大气中颗粒的大小远远大于光的波长。最著名的案例当属孚日山蓝线(Vosges blue line)[6],这一现象的发生是由于雾气中混合了树木,尤其是针叶树分泌和排出的挥发性有机物,如异戊二烯或桧烯。
最寒冷的季节,在水体(例如非常平静的湖泊或河流)的上方,通常会出现一层薄雾,如同沉积在水面上一般。值得注意的是,水体上方的雾层不会像土壤或植被上方的雾层那样迅速消失。湖面上的雾层之所以会延迟消失,是因为相比于邻近陆地,水面能够更好地反射阳光。因此,该雾层不会迅速升温,并能在较长时间内保持相对较低的温度,从而延迟雾层中小液滴的蒸发。在冬季,这一现象在法国的索恩河谷,以及大多数湖泊和池塘都十分常见。
4. 山谷处的逆温层
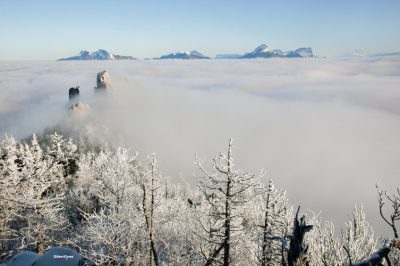
12月至3月,在冬季高压下,阿尔卑斯和比利牛斯山谷底部的气温通常会连续几天低于相同海拔的平均气温。在山谷中连续的雾层下甚至会结冰,而在1000或1500米以上的区域,在正午明媚的阳光下,温度计显示的温度却高到足以让滑雪者和徒步旅行者在户外野餐(图5)
这一异常现象是冷而重的空气从山峰附近下降到山谷造成的。这些冷气流被称为下坡风(katabatic winds)。当步行者穿过一个强风吹拂的山谷时,他们能非常真切地感觉到这种寒冷气流;这种局部寒流在当地出现的概率非常大,就像急流中的水一样流向谷底。在海拔最高处,积雪能很好地反射太阳光线,故而能将地面气温控制在相当低的范围,通常低于0℃。因此,下坡风能够毫不停歇地整日存在。在500~1000 m的海拔高度,上述条件有利于达到适宜的露点温度,也有利于水蒸气凝结,因而有利于雾的形成。徒步旅行者在更高海拔处可以俯瞰这片云海,也可以看到露出的山顶(图5)。这片云海可能会持续数日而不散。图中这种厚度不一的云层就是逆温层,在这里,温度随海拔的升高而升高,而不是像标准大气那样,随海拔的升高而降低。
与冬季相比,在夏季,高山上无积雪的草地、森林和裸岩能吸收更多的太阳辐射。在这附近,尤其是向阳坡上,从清晨开始,空气变得暖而轻,形成低压,吸引附近的空气,形成了滑翔伞爱好者所寻求的上升气流。在山谷里,阳光也加热了空气,使空气有了一定程度的扰动。接近中午时,清晨时分的下坡风将被一股名为上坡风(anabatic winds)的上升气流所取代。上坡风不同于下坡风,相对来说不容易在峡谷中聚集。上坡风的主要影响是,结合白天出现的湍流扰动,将夜晚形成的冷空气带出山谷,从而抑制逆温层的形成。
环绕山谷的山坡具有不同的朝向,这意味着在不同的山坡上,下坡风和上坡风的交接时间也不同。此外,这些山坡的具体坡度也有所差别,一侧可能较缓,而另一侧可能较陡。当山谷变宽时,会产生方向和速度均不同的水平风,进而可能导致山谷中的空气分层(详见《自然环境中流体的分层和不稳定性》)。
5. 逆温层的其他例子

[来源:https://www.meteocontact.fr/pour-aller-plus-loin/les-inversions-thermiques]
当大量暖湿气流进入某一地区时,其与初始存在的较冷空气的交汇处称为暖锋。热空气比冷空气轻,图6为温暖气流过境的示意图。这一锋面催生了一个逆温层,该逆温层将在暖湿气流前进的过程中逐渐消失。该现象被称为锋面逆温层(frontal inversion layer)。
对流层顶位于对流层的上方,海拔高度约为12 km,是一个非常特殊的逆温层,其特征是在平流层温度随海拔上升逐渐升高之前,对流层温度将随海拔升高而逐渐停止下降。这里是对流层和平流层之间的过渡区,对流层的密度较大,可以通过对流运动向上输送热量,而平流层的空气过于稀薄,无法通过对流很好地输送热量(详见《地球的大气层和气体层》)。平流层中吸收的能量大部分来自于太阳的紫外线辐射,在这里会发生氧气(O2)转化为臭氧(O3)的光化学反应,该化学反应将对外释放热量,导致温度再次上升。从对流顶层到海拔高度约50 km处,空气温度将从-56℃左右上升至接近0℃左右。
6. 对声波和电磁波的影响
与许多物质介质一样,大气具有允许这两类波传播的特性:
- 声波源于空气的可压缩性,以340 m/s的速度传播(详见《声音的产生、传播和感知》),
- 电磁波源于电磁场的振荡,以300000 km/s的光速传播(详见《黑体的热辐射》)。
尽管由截然不同的物理现象产生,但两类波对空气密度的变化都很敏感,并会被吸引到密度最高处,即最冷的地方。天气好的时候,接近地面的气层在夜间因为冷却而变得更重,因此这些波的传播路径将向地面略微弯曲,致使它们在清晨的传播范围明显大于中午。
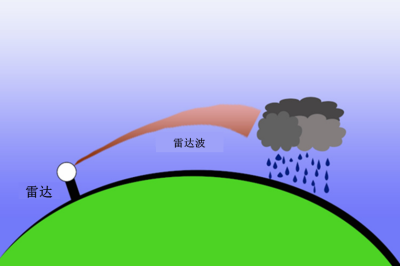
[来源:Superrefraction.jpg: LordWilson 衍生作品: Pierre cb,公共领域,维基百科共享资源]
波的传播路径主要取决于其折射率(index of refraction),就像空气密度的变化一样,折射率的变化与温度成反比,但与湿度成正比。因此,在法国,当一个温暖干燥的气团(如来自撒哈拉沙漠的气团)经过一个寒冷潮湿的气团(如来自地中海的气团)时,空气密度和波的折射率都会发生巨大的变化。在这种情况下,声波和电磁波的路径都将向地面倾斜,且曲率与地球球面曲率相同。这两类波之间的类比到此就乏善可陈了,因为它们的传播距离存在很大的差异:声波大概能传播几百米,光波可以传播几千米,微波,尤其是雷达波[7]的传播距离最长,能够达到数百公里或数千公里。
在静止的空气中,声波从声源处向四面八方传播,在途中,空气粘度会造成能量耗散,声波的能量也会分散至更大的空间中,导致声音强度逐渐减弱。当逆温层靠近地面时,声波在上升途中会逐渐穿过密度不断降低低的热空气层。这会引发折射,使声波的传播路径弯曲到水平方向。如果逆温非常明显,那么最初向上传播的声波会返回至水平方向,并与水平传播的声波汇合。尽管空气粘性的耗散依然存在,但能量的集中依然增加了声波在水平方向的传播范围。因此,当地面存在逆温层时,人的声音会传播得比没有逆温层时更远。
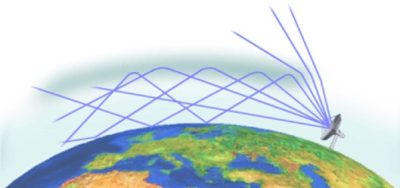
[来源:https://www.radartutorial.eu/07.waves/wa17.fr.html]
电磁波的波长范围极广,伽马射线的波长约为10-12 m,X射线的波长约为10-9 m,可见光的波长约为1 μm(10-6 m),无线电和雷达的波长则为数十米或数百米。如图7所示,雷达波是由特别设计的系统发射的,因此比声波具有更好的方向性。这些雷达发射器通常位于离地面相当高的高度,发射器位于发射塔顶部,而发射塔本身又可能设在建筑物的顶部、山岗或高山上。
因此,雷达发射出的电磁波在主方向周围形成相对通道化的波束,并利用反射的回波来探测远处的物体。然而,当存在逆温层时,雷达波也会发生折射。从图7中可以看出,逆温层使波束发生弯曲,使得探测到地平线以下的降水成为可能。当波束曲率非常接近地球曲率时,会引发波导效应[8]。当逆温层位于相当高的海拔(500 m至1500 m)时,这种效应尤为明显。这些波束被逆温层向下折射后,又被地面向上反射,因而能够会传播非常远的距离(图8)。
7. 光的特殊情况

[来源:https://fr.wikipedia.org/wiki/Rayon_vert](免费版权)
这种折射现象也适用于光,即电磁辐射的可见光波段,其波长在0.4-0.8 μm之间。正是这种现象能够让人在日落时观察到一缕绿色的光线,这是一种罕见的光学现象。这种现象具体表现为地平线上一个扁平的微小绿点,仅会在西沉的太阳上方出现几秒,作为擦过地平线的最后一束光,传播给遥远的观测者(图9)
这种绿色光线是两种不同物理现象结合的产物,可以将其他颜色(或者波长)的光从可见光波段中移除。

[来源:L’air et l’eau, EDP sciences, collection Grenoble Sciences, 2013]
当太阳即将从地平线上消失时,太阳辐射中的红色波段(波长在0.6 μm以上)在空气中的折射角大于绿色和蓝色波段(波长在0.5 μm以下),因此红色光线将不会到达千里之外的观测者。相反,经过大气折射后,蓝色和绿色光线的传播路径与地球的曲率更加接近。另一方面,瑞利散射(Rayleigh Scattering)(详见《天空的颜色》)将光谱中的蓝色部分散射到各个方向,这就减弱了直接从太阳射向观测者的蓝光的比例。因此,在黄昏前的最后几秒钟,一束波长接近0.55 μm(即绿光波长)的窄光带仍能到达遥远的观测者,但这种情况非常短暂,也非常罕见。
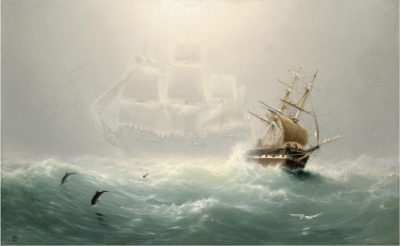
[来源:Charles Temple Dix,公共领域,维基百科共享资源]
上蜃景(superior mirage)[9],指的是物体可以在高于其自身高度的地方被观测到,该现象的形成是近地面逆温层折射现象的另一个例证。因此,这些海市蜃楼(又称蜃景)通常被称为冷海市蜃楼。例如,在尼斯的黎明,人们可以看到科西嘉山脉,但科西嘉山脉的最高峰蒙特辛托(Monte Cinto)位于尼斯的地平线之下,这是光线遵循地球曲率而出现的折射现象的另一个佐证(图10)。当逆温层在白天因为加热而消失时,蜃景也会随之消失。其他一些冷海市蜃楼的例子已经成为故事题材乃至神话传说[10],比如1860年左右由查尔斯·坦普尔·迪克斯(Charles Temple Dix)绘制的幽灵船(图11)。这是一种位于寒冷地面逆温层中的光线折射现象,可以在冰盖或南极大陆上观察到。
8.总结
- 无论是夜间的冷却,还是下坡风从高空带来冷空气,地面的冷却都能够使得地面或水体上方的温度低于标准大气温度。逆温层位于空气温度恢复到与标准大气一致(即随高度线性减小)的高度以下。
- 在冬季,这种冷却会使低层大气温度达到露点,并使该层大气中的水蒸气凝结,从而形成雾。
- 逆温层下部比上部重,空气非常稳定,没有对流运动和湍流。它就像一个盖子,阻止空气的更新和污染物的扩散。
- 当暖锋到达并经过已经存在的冷空气团时,逆温层也可能出现在对流层中层,称为锋面逆温层。
- 逆温层具有显著的特性,可以很好地传导声波和电磁波,增加它们的传播范围。罕见而短暂的绿色光线和冷海市蜃楼就是该原理的奇妙例证。
感谢CEA-LETI系统部前负责人罗兰·布兰潘(Roland Blanpain)、CEA-LETI-DSYS天线传播和感应耦合实验室负责人克里斯托夫·德拉维奥德(Christophe Delaveaud)、LEGI主任乔·索梅里亚(Joël Sommeria)和CNRM前主任菲利普·布劳特(Philippe Bougeault)对本文的校对与评论。
参考资料及说明
封面图片:半山处的逆温层和雾气。[图片来源:© DivertiCimes]
[1] 然而,这种线性下降仅限于对流层,因为在平流层(12000 m以上),吸收紫外线能量等其他机制也会发挥作用,从而导致温度升高。
[2] 露点是空气中的水蒸气能够凝结成露水或霜的温度。在任何温度下,空气所能容纳的水蒸气都有一个最大值,称为水蒸气饱和压力,在该限度外的任何多余水分子都将凝结成水滴。
[3] 气溶胶是悬浮在气体介质中的微小固体颗粒或(和)液滴的集合。典型的例子是悬浮在薄雾中的水滴。
[4] 任何气体被压缩后温度都会上升。这是因为压力和温度在宏观尺度上表现出的是同一种现象,即分子振荡(详见《压强、温度和热量》)。自行车泵在压缩过程中会发热就是该原理的典型例证。
[5] Gustav Mie, Beiträge zur Optik trüben Medien, speziell koloidaller Metallösungen, Annalen der Physik, Leipzig, vol. 25, 1908,pp. 377-445
[6] “孚日山蓝线”这一名称由朱尔斯·费里(Jules Ferry)首次提出,他于1893年在遗嘱中使用了该说法。这一物理现象同样会在在孚日山以外的地区发生。
[7] 雷达是无线电探测和测距(Radio Detection And Ranging)的缩写。它是指使用电磁波检测远处目标物体及其速度的系统,这些目标物体能够将类似于声波回波的信号反射给发射器。距离与信号的往返时间成正比。
[8] 波导是一种用来将电磁波导入特定介质(如光纤)的系统,以避免其色散,从而尽可能延长其传播路径。该过程通常利用不同介质之间的折射率变化,将发散的光线带回通道。
[9] 相比之下,低蜃境或热海市蜃楼是由于地面上存在一层特别热的空气,人们因此可以看到位于该层之上的物体景象。热海市蜃楼通常发生在沙漠或阳光充足的道路上。
[10] John MacDonald, Travels in various part of Europe, Asia and Africa during a serie of thirty years and upward, Forbes, 1790.
环境百科全书由环境和能源百科全书协会出版 (www.a3e.fr),该协会与格勒诺布尔阿尔卑斯大学和格勒诺布尔INP有合同关系,并由法国科学院赞助。
引用这篇文章: MOREAU René (2024年2月24日), 逆温层、雾和低层大气的其他奇特现象, 环境百科全书,咨询于 2024年4月26日 [在线ISSN 2555-0950]网址: https://www.encyclopedie-environnement.org/zh/air-zh/inversion-layer-fog-and-other-curiosities-of-the-lower-atmosphere/.
环境百科全书中的文章是根据知识共享BY-NC-SA许可条款提供的,该许可授权复制的条件是:引用来源,不作商业使用,共享相同的初始条件,并且在每次重复使用或分发时复制知识共享BY-NC-SA许可声明。