Lichens, surprising pioneering organisms
PDF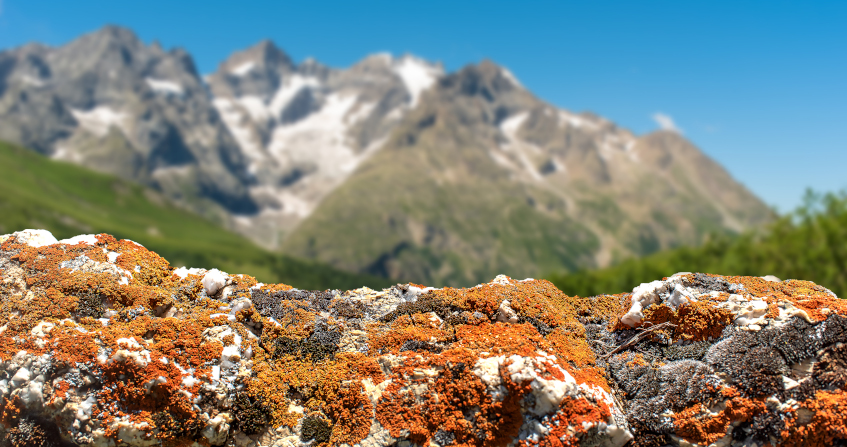
Remarkable pioneers, lichens have conquered the most extreme environments. They are able to grow on the rocks of alpine peaks or those of rocky coasts swept by the spray, on the lava flows barely cooled, hung on the branches of trees in tropical forests but also on the roof tiles of houses or the stones of our old buildings! The lichen flora of our planet represents a great biodiversity with nearly 20000 species. Beyond the diversity of shapes and colours of lichens, their ability to withstand extreme conditions is a constant source of interest for scientists.
1. Lichens, various shapes and colour palettes in landscapes
As for the shapes, there is nothing more varied. Unlike higher plants, lichens have no roots, stems or leaves. Their vegetative apparatus, called thallus, is characterized by a great diversity of shapes and more or less complex aspects, fixed in various way on the most diverse substrates (Figure 1):
- Some thalli are leaf or flake-shaped, others are more or less branched – upright or hanging – and still others are crust-shaped, very adherent to the substrate;
- Thalli are more or less firmly attached to a wide variety of substrates: tree trunks and branches, rocks and cliff walls (limestone or siliceous), ancient monuments, soils, roadsides, etc.;
- Depending on the species, the contact area between lichens and their substrate may cover almost the entire thallus or can be reduced to a single point.
2. Lichen symbiosis: an original combination
2.1. How do symbiosis works in a lichen?
“A lichen is the composite association of a fungus and a photosynthetic symbiont that results in a stable vegetative organism with a specific structure” (definition of a lichen by the International Association for Lichenology).
Studies conducted on pure symbiont cultures, then on the cultures of the two components together to carry out the synthesis, provided information on the physiology of the partners and their nutritional exchanges.
Using small filaments acting as roots, called the rhizinae, the fungus fixes the lichen to the substrate. In addition, due to its high biomass, it plays a protective role for the photosymbiont. Heterotrophic, the fungus provides the photosymbiont with water, mineral salts and certain vitamins such as vitamin C [1].
In the case of symbioses where algae are replaced by cyanobacteria, the latter form glucose (Figure 2B). The fungus transforms polyols and glucose into mannitol* and arabitol*. In addition, cyanobacteria have the ability to fix the atmospheric nitrogen (see Plants that live on Air) that is released to the fungus as ammonium.
The combination of fungus and algae is very original: the fungus produces a large number of secondary metabolites (lichen substances or lichen acids), which is not the case with the fungus alone. More than 700 molecules have been discovered. [3] These compounds provide lichens with various specific properties: fixation on the substrate, maintenance of water balance, regulation of photosynthesis, protection against light radiation or temperature variations. These secondary metabolites allow lichens to settle on substrates that do not have organic matter (stones, rocks, lava, etc.).
Many applications have been made possible thanks to these many substances: in perfumery [4], in the pharmaceutical and medical field (see Focus Lichens and therapeutic applications), in the manufacture of dyes [5], etc.
2.2. Surprising discoveries
The basic symbiotic fungus of a lichen is multicellular. Thus, the discovery of an additional mycosymbiont* consisting of specific unicellular basidiomycetes* in many lichen species belonging in particular to the family Parmeliaceae made the effect of a bomb in the community of lichenologists [8]. These unicellular basidiomycetes would be involved in the development of the structure of the thallus and the production of lichen secondary metabolites.
Lichen symbiosis is therefore not limited to a partnership between two or three components and the thallus can be considered as a true complex ecosystem (see Symbiosis and parasitism). Since the partners have constitutive characteristics that their non-symbiotic parents do not show, lichen symbiosis creates biodiversity: systematic biodiversity (with more than 20,000 species of lichens worldwide), morphological biodiversity (by creating new forms that do not exist in the mycosymbiont and photosymbiont as free organisms) and biochemical biodiversity (with the synthesis of lichen-specific secondary metabolites).
2.3. Consequences of symbiosis
Crust-like lichens, whose thallus can sometimes reach several tens of centimetres, would thus be centuries old. The low growth of lichens and their long life span are the basis of lichenometry, a method which, based on the knowledge of their growth rate and the measurement of the largest thalli, consists in evaluating the age of the lichens and consequently the age of the substrate [10]. In the case of Rhizocarpon geographicum, the measurement of thalli allowed to evaluate the age of glacial moraines on which this species developed at 300-400 years (Figure 4, [10]).
3. Lichens and their ecology
Lichens have colonized almost every environment from sea rocks to the highest altitudes, from arid deserts to high latitude regions. Only on the high seas, in highly polluted areas and on living animal tissues are they lacking. The development of each species requires particular ecological conditions determined by the types of supports, climatic factors and relationships with other living beings.
3.1. Types of substrates and habitats
The substrate conditions the water economy and the installation of the thallus by mechanical and physical factors (histological structure and bark porosity, rock density and heterogeneity, stability, structure, grain size and soil porosity) and by chemical factors (pH and mineral chemical composition or organic substances of the various substrates).
Some species indicate the presence of calcium carbonate (calcicolous species) in the substrate. Others are looking for a more acidic substrate, on soil, rock or bark (acidophilous species). Some species are indicative of metallic elements and develop only on this type of substrate or tolerate a certain amount of metals in soil or rock. The presence of iron on a rock is identified by the rust-coloured of the lichen thalli that cover it. The richness in soluble minerals, particularly nitrogen, is most often linked to the presence of bird droppings and favours the establishment of particular lichens (e.g. Xanthoria elegans).
3.2. Influence of climatic factors
The atmosphere is a very important set of factors because it provides some of the water, carbon dioxide and mineral salts used by lichens.
3.2.1. Water
Water plays a crucial role in the ecology of lichens because the degree of hydration of the thallus determines the fundamental functions. Some organisms can move quickly from the state of active life when wet to the state of slowed life when dry and vice-versa (reviviscence phenomenon). In the dry state, the water content is estimated at between 15 and 20% of the dry weight, while in the wet state, the content can reach 200 to 350%. Gelatinous thallus lichens, rich in mucilage [11], have the highest water contents (up to 3500% by dry weight). Soaking with liquid water takes less than 1 to 2 minutes, while absorption of steam is slower and sometimes takes several weeks. Conversely, dessication takes place very quickly.
This explains why, on highly exposed substrates, like on high altitude rocks, photosynthesis only takes place for a few hours in the morning after dew, or after a rain. Due to its high resistance, Xanthoria elegans is one of the species that can grow at the highest altitude (it is found at more than 7000 m in the Himalayas).
A number of lichens colonize small stones on the ground or live very close to the ground, on small boulders. They are subjected to very different climatic conditions from those experienced by species on the surfaces of the largest blocks, characterized in particular by greater thermal and water amplitudes and a supply of moisture by morning dew. Some species only settle on overhanging walls and are always protected from rain. Conversely, others can withstand flooding for a longer or shorter period of time and develop in fresh or marine water. Thus, on rocky coasts, the typical layering of lichen vegetation can be observed from the top of the boulders to the algae located at depth (see Biodiversity on rocky coasts: zoning and ecological relationships).
Some lichens support a certain atmospheric salinity and settle in the spray zone. Some maritime lichens on the Chilean coast are covered with a salt crust that plays a hygroscopic role by tripling the rate of water absorption.
3.2.2. Light and temperature
Work conducted at altitude was carried out on the effect of UV radiation on Cetraria islandica (Figure 8) during the summer [13]. The radiation showed no significant effect on photosynthetic pigments and on respiration measured at night. On the other hand, UVBs resulted in a significant decrease in the concentration of 8 out of 12 phenolic compounds. This means that in Cetraria islandica, phenolic compounds have considerable resistance to UV rays and explain the ability of this lichen to withstand high altitude radiations.
3.2.3. The action of the wind
It is done in two ways:
- an indirect, physiological action, which results in an increase in the rate of dehydration.
- a direct, mechanical action, which plays a role in the dissemination of thallus fragments and vegetative propagules.
A very particular example is that of some folicose soil lichens from desert to semi-desert steppes. Their photosynthetic activity can only be done in the wet state when they expose their upper cortex to light. In the dry state, they roll up on themselves. In this form, they are then easily transported and spread by the wind, hence the term “erratic” lichens.
Experiments carried out under conditions simulating those of space (vaccum, high UV radiation) have shown the extreme resistance of spores of different lichen species, particularly those of Xanthoria elegans. [14]
3.3. Relations with other living organisms
3.3.1. Relationships between lichens, and between lichens and plants
3.3.2. Action of animals and humans
Animals and humans cause the fragmentation of thalli on the ground by trampling and modifying chemically the environment as they enrich it with ammonia, nitrates, etc. Man himself plays a special role:
- by creating new substrates (walls, mortars, cement, tiles, quarry size fronts, etc.) where a specific lichen flora can settle;
- by disrupting the climate (drying out the atmosphere, increasing ambient temperature, pollution by dust or tar, etc.), but also by removing stations favourable to the establishment of lichens (forests reduction because of clearing, hedges and trees destroyed by the extension of cities, moors, dry grasslands, etc.).
The time required for the recolonization of a slab eroded to the rock by bryophytes and lichen groups can be more than a century. The transplant technique has been successfully implemented, either to replace an already extinct lichen flora (like Lobaria transplant [15]) or in anticipation of a reconstitution if trees are to be cut down.
4. Lichens, pioneer species?
4.1. How do lichens colonize new substrates?
Through their ability to adapt to living in extreme conditions, lichens can colonize substrates that are low in nutrients and are considered as true pioneers.
The dispersal of spores formed by lichen is one of the first steps in colonization (see Focus Lichens: hybrid organisms). Indeed, once mature, the lichen spores are violently ejected outside the asci and fall on the substrate. They germinate by emitting mycelial filaments that branch quickly. For a lichen to recover, the mycelium thus formed must meet an algae partner. Recognition is most often carried out using molecules such as lectins* that impregnate the cell walls. A young undifferentiated thallus is then formed, which gradually acquires the adult form and, most of the time, forms reproductive organs.
4.1.1. Rock colonization
Lichens are installed on rocks by mechanical and chemical action:
- On limestone rocks, oxalic acid from lichens promotes the dissolution of calcium carbonate, which is transform into calcium oxalate by thalli.
- On acidic rocks, lichen substances physically attack the rock by dissociating minerals.
Lava emitted during eruptions is quickly colonized by lichens. Let us mention Stereocaulon vesuvianum, a species with green algae and cyanobacteria, whose first location was found on the volcanic deposits of Vesuvius, Dyctionema pavonia, which develops for example on the flank of the Soufrière volcano in Guadeloupe.
Work carried out on quartzite* colonization in alpine environments has made it possible to understand the colonization strategy of Rhizocarpon geographicum (Figure 10) [17]. The crustose thallus of this species consists of compartments containing fungi and algae (or areolae). The fungus spores germinate by forming a black mycelium (hypothallus) that grows radially and on which an initial areolae is formed. Other areolae appear on the hypothallus and confluent. The first formed thalli can in turn confluence and give rise to whole thalli that can reach sizes of 15 cm in diameter [16].
4.1.2. Soil colonization
Observations conducted in the mountains showed that slopes initially bare for the construction of a ski slope were colonized by two organisms: a lichen, Baeomyces rufus and a moss, Pogonatum urnigerum. Baeomyces, begins to develop on the surface of the bare substrate and forms a very important mycelial felting that plays a role in stabilizing the surface soil (Figure 11) [18]. The Pogonatum moss has a different strategy and develops more deeply than the lichen. A real lichen-moss complex is created which favour the formation of a more elaborate soil [18].
In Cladonia, the basal part of the podetions* lyses, letting out mycelial filaments that gradually incorporate themselves into the earthy particles. A real lichen-soil complex is then formed, which is most often enriched with bacterial colonies and which, as in the previous case, will encourage subsequent colonization by higher plants. [19]
In some parts of the world, lichens constitute a significant part of ecosystems. While the global ratio between lichenic and phanerogamic flora* averages 0.1 to 1, it can exceed 100 in the Arctic regions. In these tundra areas or in northern temperate forests, several varieties of lichens – particularly reindeer lichen, Cladonia rangiferina – are a popular food for many wildlife (caribou – or reindeer-, moose, mouflon, etc.) and occasionally for some domestic animals (see Focus Lichen-animals interactions).
4.2. The origin of lichens and the colonization of land
Like many other living disciplines, lichenology is confronted with the question of origins. About fifty years ago, it was still thought that the first definite remains of lichens, found in Rhineland lignites and Baltic amber, dated only to the tertiary era. Thus, one of the first fossilized lichens, Anzia electra, found in Baltic amber from Germany (Tertiary, Lower Miocene, 20 Ma) already shows a small foliated thallus with narrow lobes, a spongy layer, a two-layer medulla and rhizinae.
It is certain that the lichens did not constitute themselves from the outset but underwent an evolution of which we are only beginning to know a few stages. [21]
Among the lichen constituents, cyanobacteria* appeared 3800 Ma in the first traces of terrestrial life (stromatolites) found in Australia in particular. At the origin of the chloroplasts of eukaryotic cells following an endosymbiosis (see Symbiosis and evolution: at the origin of the eukaryotic cell) they allowed plants to carry out photosynthesis.
Fungi, on the other hand, are difficult to fossilize, and the first real fungus appear in the Precambrian. Fossils already showing a possible association between a fungus and an algae have been discovered in the Precambrian of South Africa (from -2700 to -2300 Ma). Subsequently, fungi have transformed parallel to the plant world and symbiotic associations (mycorrhizae, lichens) are the result of a long evolution of interactions with photosynthetic organisms.
Ancient traces of lichens have been found in the fossiliferous formation of Dushanto (China, Guizhou Province) dating from the early Ediacaran period (635-551 Ma). These exceptionally preserved microfossils show thin filaments associated with cells that have been interpreted as cyanobacteria or green algae similar to current organisms. A probably lichenized fungus, Cosmochlaina, discovered in England in the Middle Ordovician (460 Ma) shows an intact cortex and a second cortical layer in electron microscopy. But the photosymbiont was not found. [22]
Two Lower Devonian fossils found in England, Cyanolichenomycitis devonicus and Chlorolichenomycitis salopensis, are considered the first true lichens to be found due to their structural similarity to existing lichens. Indeed, the first fossil shows a heteromeric thallus with a thick cortex on the surface and a medulla filled with cyanobacteria colonies resembling the current Nostocs. These fossils are interpreted as lichens belonging to the Pezizomycotina, with an anatomical structure similar to that of current Lecanoromycetes. [23]
The lichen symbiosis is probably much older than the proven lichen samples. The discoveries are hampered by the fact that the lichen components are difficult to fossilize and the samples found are often of poor quality. However, it is to be hoped that current investigation methods (dating, molecular biology, etc.) that continue to be improved will provide valuable information for future fossil lichen discoveries.
It seems highly probable that the colonization of the surface of rocks or soil by various types of microorganisms and lichens in appropriate climatic zones was one of the first steps in the conquest of land by living organisms during the Devonian (see The First Terrestrial Ecosystems). The current existence of biological crust gives an idea of some of the primitive forms of this colonization, but many others have been possible. Currently, existing terrestrial vegetation areas in the most extreme ecosystems (Arctic, Antarctic, Alpine, desert and steppe) are dominated by lichens wherever there is little or no competitive pressure from higher plants due to harsh environmental conditions. When they appeared, the higher plants were then able to settle on rocks and sediments partly altered by the activity of microorganisms and lichens. They then participated themselves in the formation of a soil, on which they were able to root and develop and which they feed with their own organic debris.
5. Messages to remember
- Lichens grow in all environments except the high seas, on the tissues of live animals and in highly polluted areas.
- Lichen symbiosis is more complex than previously thought: the structure of the thallus shows not only the presence of two basic partners (a fungus + a green algae and/or a cyanobacterium) but also that of many bacteria and a unicellular fungus recently discovered.
- Lichens are reviviscent: after desiccation, they are able to return to active living as soon as they are rehydrated.
- In the presence of algae only, the fungus produces lichen substances that facilitate the fixation of lichen on the substrate and help to protect it.
- Lichens grow very slowly and some lichens can live for several centuries.
- Lichens are pioneers: they have the ability to settle on substrates that are very low in nutrients and can withstand extreme temperature and light conditions.
- The first known lichens date back to the Devonian.
Notes and references
Cover image. Rock covered with Xanthoria elegans (foreground) in the Lautaret alpine garden; the Meije (3 984 m) is in the background [Source: Photo © J. Joyard & M. Neuburger].
[1] Sometimes, the fungus can behave as a saprophyte by pulling organic substances from the environment or live as a parasite on another lichen.
[2] Present throughout the plant world, fungi, lichens and many algae, polyols are derived from oses. About 30% of primary carbon production on earth is through the synthesis of polyols in plants and algae. In plants, polyols are involved in tolerance to abiotic and biotic stresses and are a form of transport and storage of carbon skeletons.
[3] Some of these lichen metabolites are toxic. For example, in Letharia vulpina – an altitude cortex species recognizable by its mustard-yellow colour and growing mainly on larch and cembro pine – the vulpinic acid present gives lichen its toxicity. Letharia vulpina was once used as a mixture with bait to make wolves and foxes disappear. However, it should be noted that the effects were reinforced by the introduction of crushed glass into the bait.
[4] Lichens, already known as perfume fixatives since the Middle Ages, are still widely used in perfumery today. Two corticulose species, Pseudevernia furfuracea, Evernia prunastri (incorrectly called “oak moss”), which owe their fixing properties to their high atranorin content, are harvested in large quantities, mainly in Central Europe and imported into Grasse (France). Local perfumeries extract a concentrate called “absolute oak moss” which is used to make many perfumes. Each year, 6,000 to 8,000 tons of lichens are harvested in southern France, Morocco and other countries. These intensive removals threaten the survival of the species. Ideally, they should be replaced by synthetic products, but so far no one has been able to reproduce their complex biochemical nature.
[5] Since ancient times, various dyes have been extracted from lichens, such as the orseilles from the Roccella, which give red hues. Other lichens give brown to red tones (Umbilicaria pustulata), yellow tones (Letharia vulpina, Flavoparmelia caperata), orange yellow to pink tones (Xanthoria parietina…), green (various Cladonia)… Remember that the “sunflower” liqueur which turns red or blue according to the acidity or basicity of the solution to be tested is extracted from different lichens (Roccella, Dendrographa…). Despite the use of chemical dyes, some Irish tweeds are still coloured with lichens.
[6] Grube, M., Cernava, T. Soh , J., Fuchs, S., Aschenbrenner, I., Lassek, C., Wegner, U., Becher, D., Riedel, K., Sensen, C.W. & Berg, G. 2015. Exploring functional contexts of symbiotic sustain within lichen-associated bacteria by comparative omics. ISME J. , 9, 412–424.
[7] This great diversity of bacteria associated with lichens and their essential functions in lichen symbiosis suggests that the concept of microbiota (a term that refers to all bacteria living in and with living organisms) can very logically be extended to lichens.
[8] Spribille, V. Tuovinen, P. Resl, D. Vanderpool, H. Wolinski, M.-C. Aime, K. Schneider, E. Stabenheimer, M. Toome-Heller, G. Thor, H. Mayrhofer, H. Johannesson & McCutcheon, J.-P. 2016. Basidiomycete yeasts in the cortex of ascomycete macrolichens. Science, 353, (6298), 488-492.
[9] In contrast, foliose and fruticose lichens grow faster: a Ramalina holds the record with an annual growth of 10 cm.
[10] Beschel, R. 1957. Lichenometrie im Gletschervorfeld. Jahrbuch des Vereins zur Schutze des Alpenflora und Tiere. 22p.
[11] Mucilages are plant substances, made up of polysaccharides, which swell on contact with water to a viscous gelatin-like consistency.
[12] Aubert, S., Juge, C., Boisson, A.-M., Gout, E. & Bligny, R. 2007. Metabolic processes sustaining the reviviscence of lichen Xanthoria elegans (Link) in high mountain environments. Planta, 226, 1287-1297.
[13] Bachereau, F. & Asta, J., 1997. Effects of solar ultraviolet radiation at high altitude on the physiology and the biochemistry of a terricolous lichen Cetraria islandica (L.) Ach. Symbiosis, 197-217; Bachereau, F. & Asta, J., 1998. Effects of solar ultraviolet radiation at high altitude on the phenolic compounds contents of Cetraria islandica (L.) Ach. Ecologie, 29(1-2), 267-270.
[14] Vera J.P., Horneck, G., Rettberg, P. & Ott, S. 2004. The potential of the lichen symbiosis to cope with the extreme conditions of outer space II : germination capacity of lichen ascospores in response to simulated space conditions. Adv. Space Res. 33, 8, 1236-1243.
[15] Scheidegger, C. (1995). Early development of transplanted isidioid soredia of Lobaria pulmonaria in an endangered population. Lichenologist, 27(5), 361-374.
[16] Galsomiès, L., Robett, M. & Orial, G. 1999. Interaction lichens-roche sur monument historique en granite. Bull. Inf. As. Fr. Lichenol. Mémoire n° 3, Grenoble, 35-42.
[17] Asta, J. & Letrouit, M.A. 1995. Observations on the early growth of Rhizocarpon geographicum thalli. Herzogia, 11, 229-252.
[18] Asta, A. et Souchier, B. 1999. Lichens et pédogenèse : dynamique de la végétation et études micromorphologiques de l’interface-lichen-sol. Bull. Inf. As. Fr. Lichénol. Mémoires n°3, 29-34.
[19] Asta, J., Orry, F., Toutain, F., Souchier, B. & Villemin, G. 2001. Micromorphological and ultastructural investigations of the lichen-soil interface. Soil Biol. Biochem. 33, 323-337.
[20] Scheidegger, C. 2015. Touche pas à ma croûte ! Année Internationale des sols. Fiche_croute_biologique.pdf
[21] Farou, J.L. 2017. Sur la piste des premiers lichens. Bull. Inf. As. Fr. Lichénol., 42, 2, 240-248.
[22] Edwards, D., Axe, L. & Honegger, R. 2013. Contributions to the diversity in cryptogamic covers in the mid-Paleozoic: Nemato thallus revisited. Bot. J. Linn. Soc. 173, 505-534.
[23] Honegger, R., Edwards, D. & Axe, L. 2013. The earliest records of internally stratified cyanobacterial and algal lichens from the Lower devonian of the Welsh Boerderland. New Phytol., 197, 264-275.
The Encyclopedia of the Environment by the Association des Encyclopédies de l'Environnement et de l'Énergie (www.a3e.fr), contractually linked to the University of Grenoble Alpes and Grenoble INP, and sponsored by the French Academy of Sciences.
To cite this article: ASTA Juliette (August 21, 2019), Lichens, surprising pioneering organisms, Encyclopedia of the Environment, Accessed July 27, 2024 [online ISSN 2555-0950] url : https://www.encyclopedie-environnement.org/en/life/lichens-pioneering-organisms/.
The articles in the Encyclopedia of the Environment are made available under the terms of the Creative Commons BY-NC-SA license, which authorizes reproduction subject to: citing the source, not making commercial use of them, sharing identical initial conditions, reproducing at each reuse or distribution the mention of this Creative Commons BY-NC-SA license.